Panagiotis Tassis
Assistant Professor of Swine Medicine and Reproduction Clinic of Farm Animals, School of Veterinary Medicine, Aristotle University of Thessaloníki Greece
The significance of reproductive performance for the outcome of swine production is undoubtable. Both sides, gilts/ sows and boars are the basis of proper and financially rational production in intensive swine farms globally.
⇰ Genetic lines of hyperprolific sows, as well as high durability and increased performance boars are needed for increasing production demands and high-quality pork meat.
A variety of major issues, such as reproductive management, selection of gilts and boars, introduction of gilts to farm’s reproductive program, environmental conditions affecting reproductive performance (e.g. heat stress, etc.), along with maintenance of high health status and fulfillment of specific nutritional requirements, should be taken care during the rearing of hyperprolific sows and boars.
⇰ Controlling the major viral or bacterial swine pathogens affecting the genital tract and reproductive performance, as well as proper nutrition in terms of ingredient/ nutrient requirements and a proper feeding schedule during various stages of gestation and boar’s life, are significant parts of proper reproduction management.
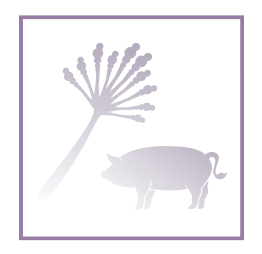
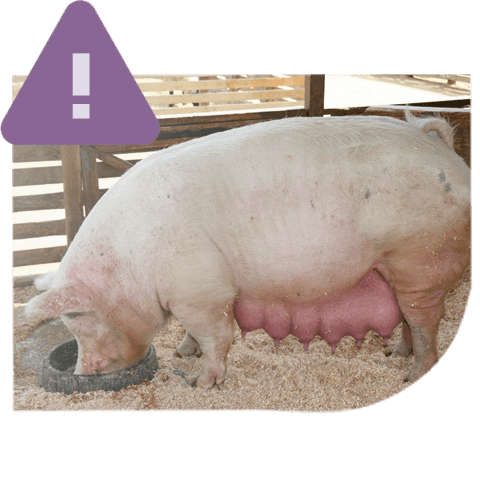
Mycotoxins are secondary metabolites of certain fungi species that can be found in grains worldwide. They are produced before (fungi as plant pathogens) or after the harvest of grains, or even during storage (fungi growing saprophytically).
In particular regions, the mycotoxin menace seems to be higher resulting in severe outbreaks of intoxication (mycotoxicosis) threatening humans and animals1.
⇰ The significant impact of mycotoxins includes loss of human and animal life, increased health care and veterinary care costs, and reduced livestock production2.
The most important mycotoxin producing fungi belong to the genera Aspergillus, Penicillium, Fusarium, Alternaria y Claviceps3.
Out of more than 500 mycotoxins, some are considered extremely significant for pig health and productivity.
Namely, aflatoxins (AF) B1, B2, G1 y G2, deoxynivalenol (DON), zearalenone (ZEN), fumonisins (FB1, FB2, FB3) and ochratoxin A (OTA) are considered significant for their devastating effects on pig production worldwide.
Other mycotoxins such as T-2 toxin, nivalenol or ergot alkaloids have been suggested as somewhat significant for swine, especially in particular geographical regions1,4.
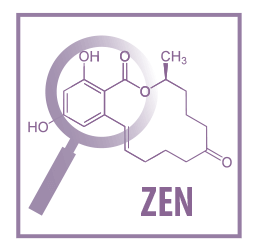
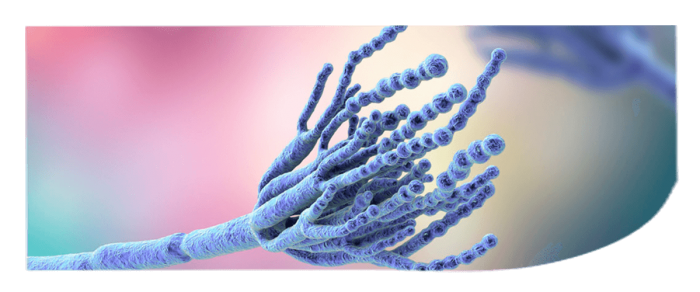
In this article, we will try to synopsize effects of the most important mycotoxins ingested separately, on the two parts of reproduction, i.e. the female part (gilts, sows and the offspring) and the male part which includes the boars.
Predominantly direct toxic effects of AF, OTA, DON, T-2, FB and ZEN are described, since presentation of indirect effects (e.g. increased susceptibility to infections due to impaired immunity that could affect reproduction, or effects of reduced protein synthesis or feed refusal on gestation and litter weight) is practically more or less extremely extensive. In vivo and in vitro effects along with aspects related to the hormonal alteration of reproduction according to relative literature are also described.
Reproductive effects of Aflatoxinas in swine
Aflatoxins (AFs) are produced mainly by Aspergillus flavus, A. parasiticus and A. nomius and are detected usually in maize, peanuts and cottonseed.
The most common AFs are AFB1, AFB2, AFG1and AFG2.
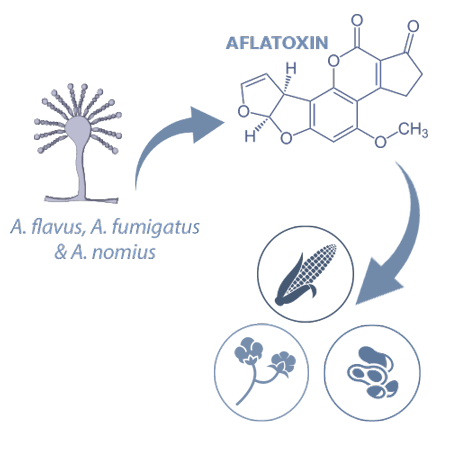
AFB1 is considered as an active hepatocarcinogen and is the most significant in terms of toxicity in swine6.
⇰ The liver is considered the primary target organ of AFB1.