Abdelhacib Kihal
DVM, MSc, PhD in Animal Production Department of Animal and Food Science, Universitat Autònoma de Barcelona, Spain
Mycotoxins represent an important challenge for animal production and their prevalence in raw materials or feedstuffs is subject to variations related to changes in climate conditions.
For example, droughts or years with increased precipitations affect the type of fungi that proliferate in certain regions (Moretti et al., 2019).
There are different methods to control the impact of mycotoxins in animal production, from controlling their presence in raw materials during pre-harvest and post-harvest, to detoxification of animals exposed to them through contaminated diets.
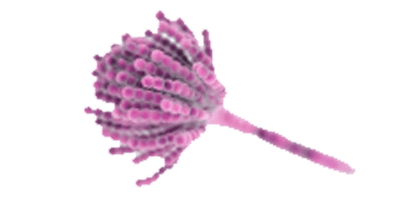

Mycotoxin adsorbents are active in the gastrointestinal tract of animals, binding mycotoxins to the adsorbent matrix through different physicochemical interactions.
There are different types of adsorbents, the most common being clays, activated carbon (AC) and yeast cell walls (YCW), whose interlaminar space, pores and β-glucans represent their key adsorption factors, respectively (Jouany, 2007).
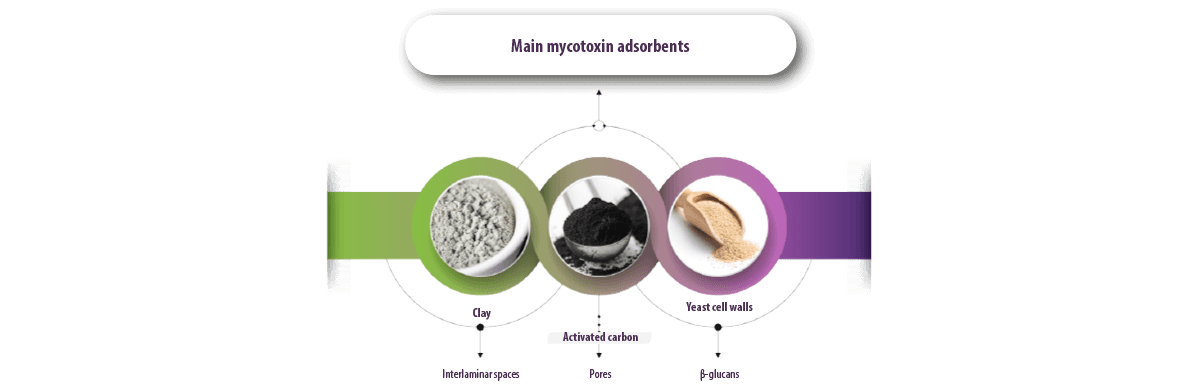
To determine the efficacy of mycotoxin adsorbents, in vitro tests are mainly used, allowing the evaluation of a wide number of adsorbents and mycotoxins with the advantage of being quick and inexpensive compared with in vivo tests.
However, there are many variants of in vitro tests that have been developed over the years in an attempt to simulate the processes of the gastrointestinal tract of animals.
The problem with these methods is the high variability of mycotoxin adsorption results.
These protocols vary in complexity, from a simple test with distilled water and incubation at room temperature (Lemke et al., 2001) to more complex methods that simulate the processes of the gastrointestinal tract of animals using different pH and including gastrointestinal enzymes, or using gastric juice as the incubation medium (Avantaggiato et al., 2004; Gallo y Masoero, 2010).
Unfortunately, this diversity of experimental protocols has resulted in high variability in the results of adsorption capacity of adsorbents (Kihal et al., 2022).
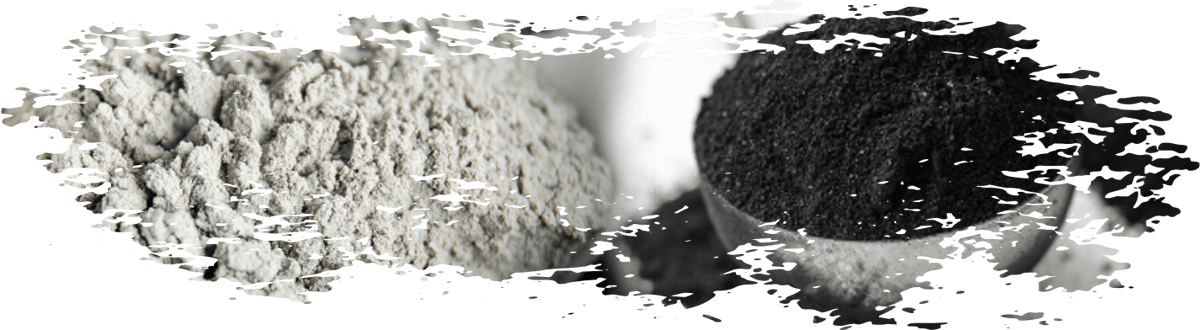
Comparative discussion on the adsorption
capacity of adsorbents in in vitro and in vivo studies
Determining mycotoxin adsorption capacity in in vivo tests is essential to demonstrate the real efficacy of the product.
In contrast to in vitro tests, in vivo tests replicate field conditions and animal response to adsorbent supplementation in the presence of mycotoxins in diets.
The key finding of this study was that the efficacy of different adsorbents [activated carbon (AC), bentonite, aluminosilicates (HSCAS), yeast cell walls (YCW), and a mixture of adsorbents (MIX)] significantly reduced the percentage of AFM1 in milk compared to the control.
⇰ These results confirm the efficacy of the adsorbents to adsorb aflatoxins from in vitro assays, although the percentage adsorption in vivo was lower. Furthermore, the percentage reduction between different adsorbents in vivo was not different, demonstrating a similar effect between the different adsorbents.
It is important to note that the number of in vivo studies that were included in this meta-analysis was lower than the number of in vitro studies (28 vs. 68 articles).
⇰ This difference may have an effect on the variability of the results leading to high variability between treatment comparisons.
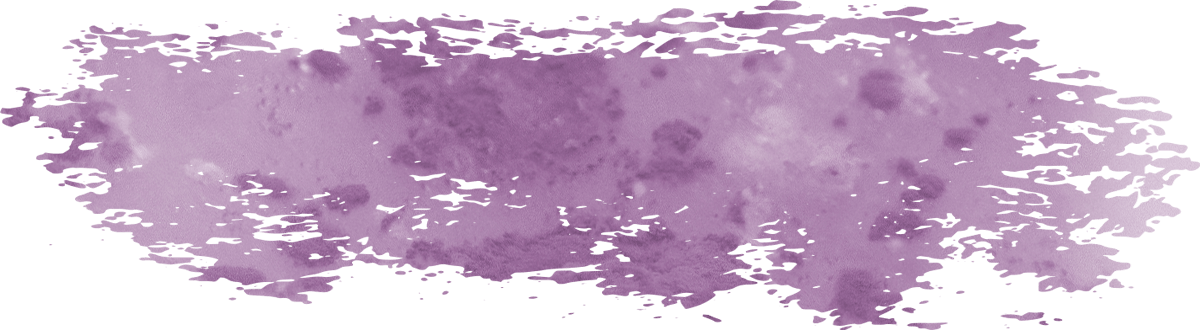
Challenges and limitations of in vivo studies
In vivo studies, unlike in vitro tests, are complicated, expensive, and difficult to implement:
- ⇰ They require the application of biosafety protocols for handling mycotoxins at the farm level.
- ⇰ The required facilities are not available at most research centers.
In addition, as with in vitro tests, in vivo studies must be performed under experimental conditions to avoid misleading results.
ADSORBENT:MYCOTOXIN RATIO
Defining the adsorbent:mycotoxin dosage is a common problem in in vitro and in vivo studies, as working with the wrong ratios can favor or disfavor mycotoxin adsorption results.
ROUTE OF EXPOSURE
The form of feed contamination in experimental work is also a key factor in the evaluation of adsorption capacity.
For example, supplementation of naturally contaminated feed can affect the results compared to supplementation of pure AFB1 directly into the cow’s rumen.
⇰ This is because naturally contaminated feed may contain different types of aflatoxins (AFB1, AFB2, AFG1 or AFG2) or other mycotoxins, resulting in synergistic phenomena or competition for binding to the tested adsorbent.
TYPE OF SUBSTRATE
The raw material used for fungal growth is very important, since fungi use nutrients from contaminated grain for their growth and using different types of grain can also affect fungal growth and mycotoxin production.
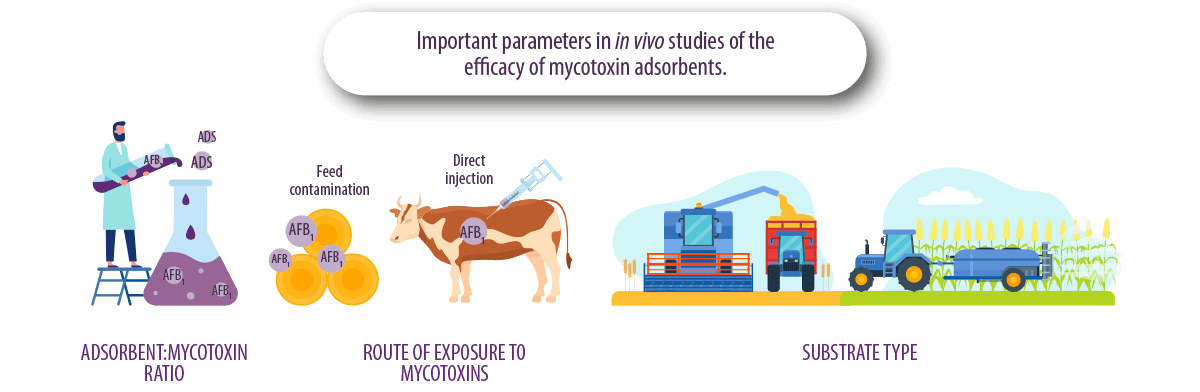
The review of the results of in vitro and in vivo studies of adsorbents (Kihal et al., 2023) confirmed that their in vitro aflatoxin adsorption capacity was similar to in vivo experiments where different adsorbents (activated carbon, bentonite and HSCAS) successfully decreased the concentration of AFM1 in milk in the range of 26-45%.
In contrast, YCW showed the lowest adsorption capacity in in vitro tests, which is consistent with in vivo tests in which YCW were the least effective adsorbents in reducing AFM1 transfer in milk.
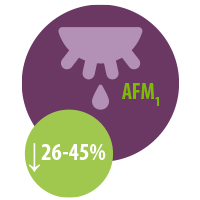
It is worth noting that the use of the adsorbents in vivo resulted in a lower adsorption capacity compared to the in vitro results, with a decrease of more than 50% for AC, bentonite and YCW, and a decrease of 67% for HSCAS

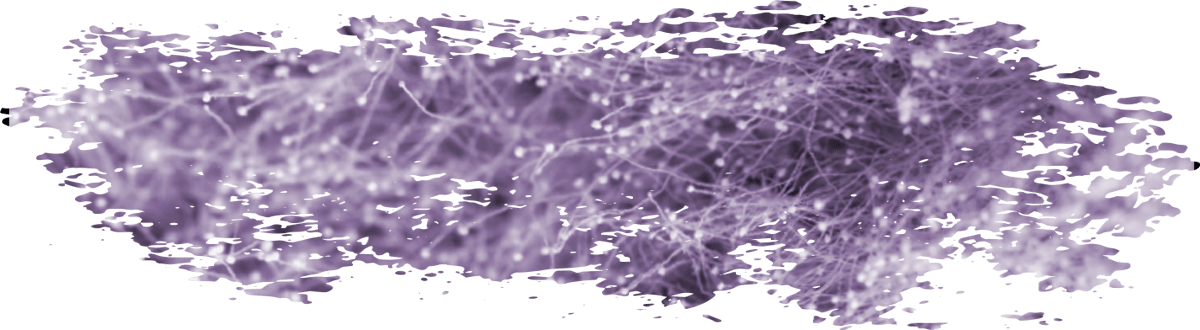
What is the potential for mycotoxin adsorbents to interfere with the adsorption of other nutrients and which is the best tool to test this, in vitro or in vivo?
Recent studies in our laboratory have shown that the adsorption capacity of mycotoxin adsorbents is affected by:
- ⇰ The type of adsorbent
- ⇰ The mycotoxin
- ⇰ The characteristics of the incubation medium
(Kihal et al., 2022)
In addition, this capacity may be altered by the interaction with nutrients present in the same environment with mycotoxins, both in vivo and in vitro.
The adsorption mechanism of adsorbents does not selectively bind only mycotoxins, but can also adsorb other molecules present in the gastrointestinal tract of the animal, such as nutrients.
This ability to bind nutrients is attributed to the physicochemical similarities of some nutrients with mycotoxins that allow their interaction.
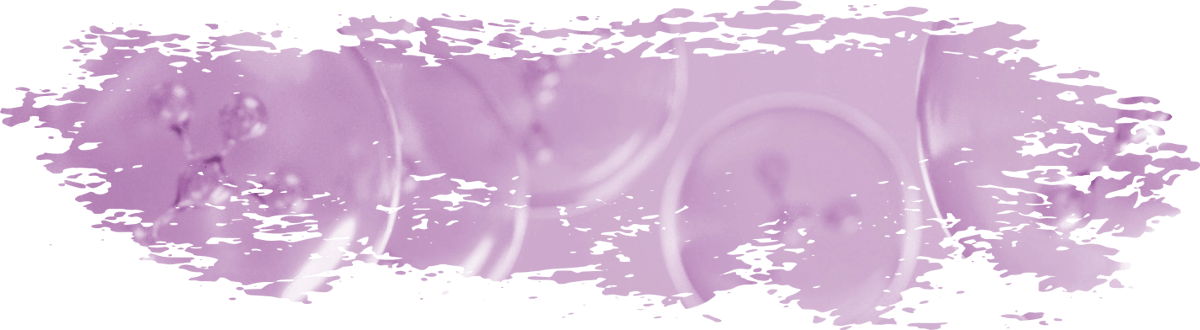
In vitro studies on the adsorption capacity of nutrients
Kihal et al. (2020; 2021) studied the interaction of six different adsorbents with amino acids and vitamins.
The authors reported an adsorption range of 27-37% for amino acids, 25-58% for water-soluble vitamins and 10-29% for fat-soluble vitamins.
Vekiru et al. (2007) also observed that CA adsorbed a large proportion of vitamin B8 (78%) and B12 (99%), while bentonite had lower adsorption of vitamin B12 (47%).
Barrientos-Velázquez et al. (2016) reported that bentonite adsorbed 34% of vitamin B1 and, in parallel, aflatoxin adsorption was reduced by 34%, indicating direct competition from other nutrients for adsorption sites.
Mortland et al. (1983) reported that smectite has the capacity to adsorb vitamin B2 (50%).
Bentonite and montmorillonite have also been observed to adsorb proteins in an in vitro model (Ralla et al., 2010; Barrientos-Velázquez et al., 2016).
The ability of adsorbents to adsorb minerals was also investigated in vitro by Tomasevic-Canovic et al. (2001) who observed a high capacity of bentonite to adsorb copper (56%) and cobalt (73%), while the adsorption of zinc (12%) and manganese (12%) was relatively low.
-
In contrast, vitamins A, D, B3, B5 and B8, and the amino acids, tryptophan and phenylalanine, were not adsorbed by bentonite and zeolite (Tomasevic-Canovic et al., 2001; Vekiru et al., 2007; Kihal et al., 2020).

⇰ The adsorption capacity of water-soluble vitamins was higher due to their low molecular weight and the presence of more than one hydroxyl or carbonyl group that ensures stable adsorption with the adsorbents.
⇰ The adsorption of vitamin D was lower due to its higher molecular weight and the presence of different ramifications that prevent its entry into the adsorption sites of the adsorbent.
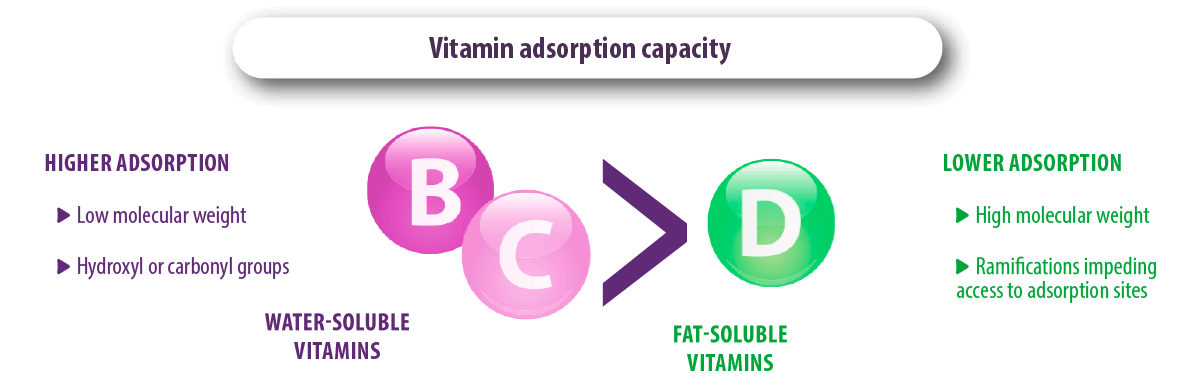
Despite these results, there are technical issues when applying these methods to some nutrients, since some of them are sensitive to environmental factors and may suffer alterations during incubation.
Kihal et al. (2021) observed that vitamin A disappeared from the incubation medium after 4 hours of incubation which does not allow its titration, while vitamins D and E were more stable during the degradation kinetics and showed more than 90% stability.
Díaz et al. (2004) pointed out that the results of in vitro tests should not be considered as final results and suggested that in vivo studies should be performed to have more reliable results.
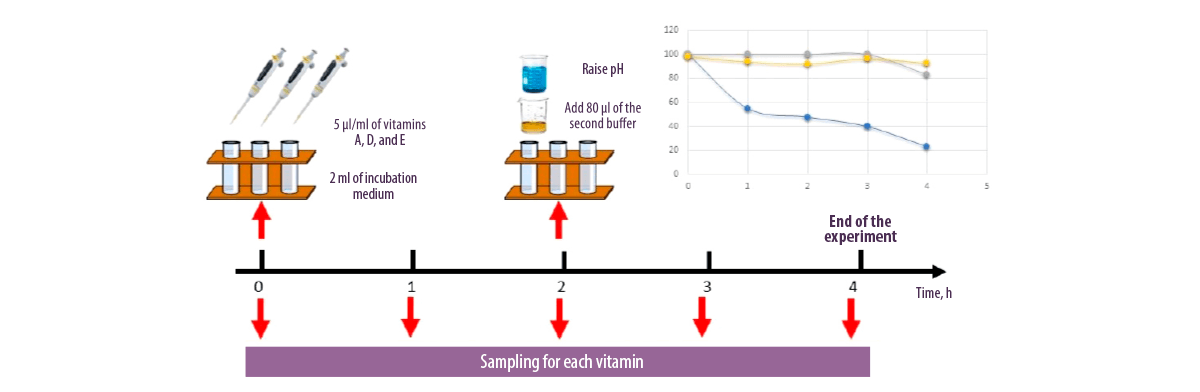
Figure 1. Evaluation of the stability of fat-soluble vitamins in the incubation medium.
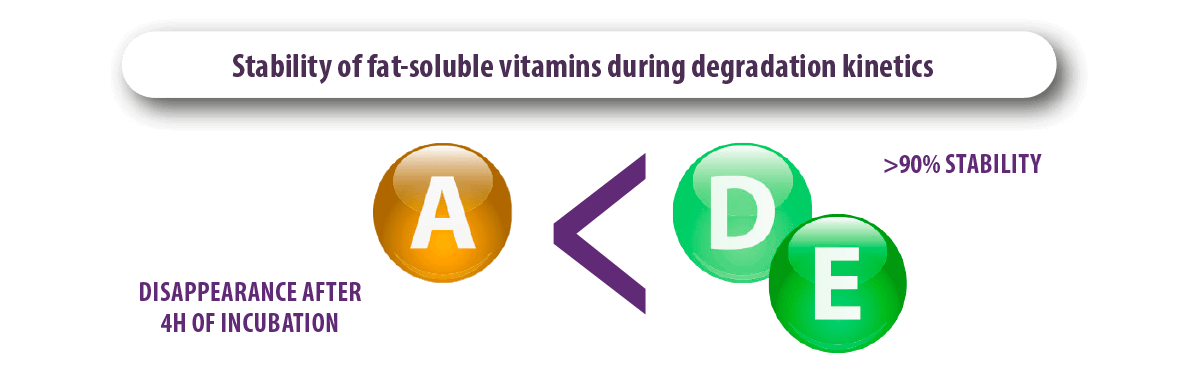
In vivo studies on the adsorption capacity of nutrients
Briggs y Fox (1956) observed that supplementation of broiler diets with 2-3% bentonite resulted in vitamin A deficiency.
Zinc content also decreased in broiler bones after they were supplemented with HSCAS from 0.5 to 1% of the diet (Chung et al., 1989).
In contrast, Afriyie-Gyawu (2004) and Pimpukdee et al. (2004) observed that the inclusion of 0.5% bentonite did not affect vitamin A concentration in the liver and Chung et al. (1989) found that the inclusion of HSCAS did not affect the bioavailability of vitamin A, vitamin B2 and manganese in chicks.
Sulzberger et al. (2016) and Kihal et al. (2022), after supplementing dairy cows with 1.2 and 2% montmorillonite in the dairy cow diet, also observed no changes in the plasma concentration of vitamins A, D, E, B1 and B6.
Maki et al. (2016) supplemented HSCAS to dairy cows at 1.2% of the dietary dry matter and observed no negative effects on the bioavailability of vitamins A and B2 in milk.
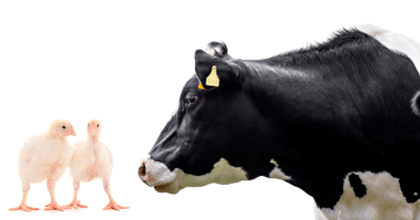

Recommendations for improving the accuracy of in vitro and in vivo tests
Standardized adsorbent:mycotoxin ratios
In addition to the factors limiting the specific interaction between adsorbents and mycotoxins, the adsorption mechanism of adsorbents is saturable and depends on the number of adsorption sites available for mycotoxins in the matrix.
For this reason, the ratio of adsorbent:mycotoxin is an essential factor in in vitro tests where the adsorption capacity can be easily manipulated:
⇰ High adsorbent concentration and low mycotoxin concentration: incubation of a high adsorbent concentration with a low mycotoxin concentration will lead to a higher adsorption capacity of the tested adsorbents because there are more adsorption sites available than mycotoxins present in the medium.
⇰ Low adsorbent concentration and high mycotoxin
concentration: incubation of a low adsorbent dose with a high mycotoxin dose will lead to a lower adsorption capacity due to saturation of the available adsorption sites on the adsorbents.
(Sulzberger et al., 2017)
Using the data selected for in vitro adsorbent efficacy analysis, the adsorbent:mycotoxin ratios used in the current techniques resulted in a very wide range of ratios regardless of the type of mycotoxin or adsorbent (1:0,00007 to 1:600 mg/μg, Table 1).
Table 1. Ranges of the ratio of mycotoxin and mycotoxin adsorbents (mg:μg) used in in vitro tests to determine the adsorption capacity of different mycotoxins and mycotoxin adsorbents.

⇰ La EFSA (2017) considers adsorbents to be safe and sets high safety limit doses (20 kg/t feed).
The currently recommended doses of adsorbent are generally established by the adsorbent marketing companies that perform in vitro tests using different doses of adsorbent inclusion.
This required dosage differs according to the type of adsorbent, changing chemical properties with the chemical composition and nature of the adsorbent. However, it should be possible to recommend a range of ratios that should be followed during in vitro experiments.
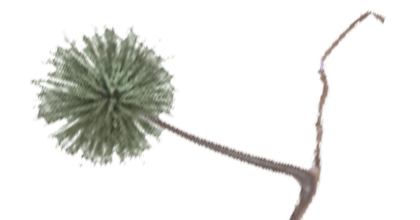
To standardize an in vitro protocol, we propose to use an adsorbent:mycotoxin ratio close to that found in field conditions.
- ⇰ For this purpose, the daily intake of adsorbents and mycotoxins should be determined.
- ⇰ The practical dose of adsorbents should be considered based on what has been shown to be effective in adsorbing mycotoxins.
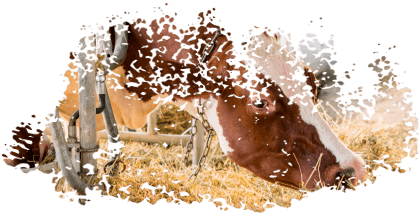
Díaz et al. (2004) recommended a 1.2% daily dry matter adsorbent concentration equivalent to 300 g/day/cow. However, the daily intake of mycotoxins is very variable and depends on the type of mycotoxin.
⇰ Therefore, it is reasonable to propose an adequate dose related to the minimum toxic levels of each mycotoxin evaluated by the European Commission (EC, 2006 on raw materials).
Since mycotoxicosis occurs in animals with high mycotoxin concentrations, we propose to use the minimum toxic concentration for each mycotoxin multiplied by 10 for in vitro tests.
⇰ Then, the daily intake will be 10 times higher than the minimum toxic limits considering an average consumption for each animal species.
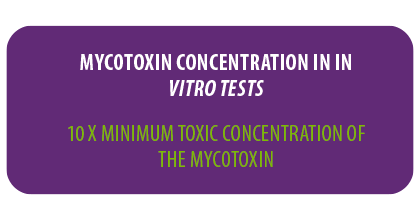

Standardization of in vitro tests
A standardized procedure must also consider other aspects, such as the characteristics and volume of incubation media, duration, and pH, among others.
In vitro testing validation process
Finally, like any in vitro test, validation should be necessary.
However, it is very difficult to perform enough in vivo tests to provide sufficient data for each adsorbent and each mycotoxin for the validation process, which increases the difficulty of developing a reliable and validated test.
CONCLUSIONS
Due to the variability in the results and the limited data available, it is important to:
- 1. Standardize an in vitro method to evaluate the ability of adsorbents to adsorb mycotoxins and other nutrients in vitro.
- 2. Validate the results with in vivo tests.
The actual in vitro protocols used to evaluate adsorbents are designed as a screening method and are mainly used during product development because they provide quick and inexpensive information on the efficacy of the products. However, this information is limited due to high variability between methods and laboratories.
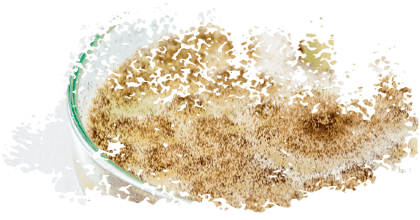
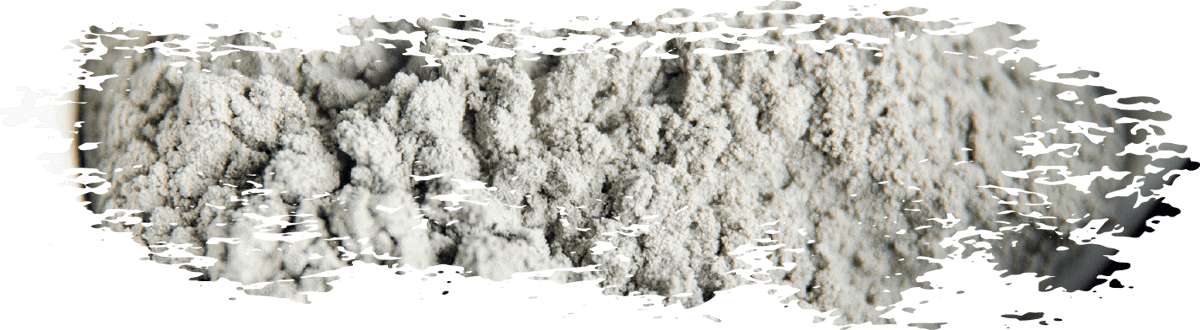
BIBLIOGRAPHY
Afriyie-Gyawu, E. 2004. Safety and efficacy of Novasil clay as a dietary supplement to prevent aflatoxicosis. Ph.D. Diss. Texas A&M University, Texas.
Avantaggiato, G., R. Havenaar, and A. Visconti. 2004. Evaluation of the intestinal absorption of deoxynivalenol and nivalenol by an in vitro gastrointestinal model, and the binding efficacy of activated carbon and other adsorbent materials. Food Chem. Toxicol. 42:817–24. doi:10.1016/j.fct.2004.01.004
Barrientos-Velázquez, A. L., S. Arteaga, J. B. Dixon, and Y. Deng. 2016. The effect of pH, pepsin, exchange cation, and vitamins on aflatoxin adsorption on smectite in simulated gastric fluids. Appl. Clay Sci. 120:17-23. doi:10.1016/j.clay.2015.11.014
Briggs, G. M., and M. R. S. Fox. 1956. Vitamin a deficiency in chicks produced by adding high levels of bentonite to synthetic diet. Poult. Sci. 35:570-576. doi:10.3382/ps.0350570
Chung, T. K., J. W. Erdman, and D. H. Baker. 1990. Hydrated sodium calcium aluminosilicate: effects on zinc, manganese, vitamin A and riboflavin utilization. Poult. Sci. 69:1364-1370. doi:10.3382/ps.0691364
Diaz, D. E., W. M. Hagler, J. T. Jr. Blackwelder, J. A. Eve, B. A. Hopkins, K. L. Anderson, F. T. Jones, and L. W. Whitlow. 2004. Aflatoxin binders II: Reduction of aflatoxin M1 in milk by sequestering agents of cows consuming aflatoxin in feed. Mycopathol. 157:233-241. doi:10.1023/B:MYCO.0000020587.93872.59.
European Commission (EC), 2006. EC regulation No 401/2006. Laying down the methods of sampling and analysis for the official control of the levels of mycotoxins in foodstuffs. Off. J. Europ. Comm. L 70: 12-34.
European Food Safety Authority (EFSA). 2017. Safety and efficacy of bentonite as a feed additive for all animal species. EFSA J. 15:5096. https://doi.org/10.2903/j.efsa.2017.5096
Gallo, A., and F. Masoero. 2010. In vitro models to evaluate the capacity of different sequestering agents to adsorb aflatoxins. Ital. J. Anim Sci. 9:1, e21. doi:10.4081/ijas.2010.e21
Galvano, F., A. Piva, A. Ritieni, and G. Galvano. 2001. Dietary strategies to counteract the effect of mycotoxins: A review. J. Food Prot. 64:120-131. doi:10.4315/0362-028X-64.1.120
Jouany, J. P. 2007. Methods for preventing, decontaminating and minimizing the toxicity of mycotoxins in feeds. Anim. Feed. Sci. Technol. 137:342-362. doi:10.1016/j.anifeedsci.2007.06.009
Kihal, A., M. E. Rodríguez-Prado, and S. Calsamiglia. 2022. A network meta-analysis on the efficacy of different mycotoxin binders to reduce aflatoxin M1 in milk after aflatoxin B1 challenge in dairy cows. J. Dairy Sci. In press.
Kihal, A., M. E. Rodríguez-Prado, and S. Calsamiglia. 2022. The efficacy of mycotoxin binders to control mycotoxins in feeds and the potential risk of interactions with nutrient: a review. J. Anim. Sci. 100:1-14. doi:10.1093/jas/skac328
Kihal, A., M. E. Rodríguez-Prado, C. Cristofol, and S. Calsamiglia. 2021. Short communication: Quantification of the effect of mycotoxin binders on the bioavailability of fat-soluble vitamins in vitro. Animals. 11:2251. doi:10.3390/ani11082251
Kihal, A., M. E. Rodriguez-Prado, C. Godoy, C. Cristofol, and S. Calsamiglia. 2020. In vitro assessment of the capacity of certain mycotoxin binders to adsorb some amino acids and water-soluble vitamins. J. Dairy Sci. 103:3125–3132. doi:10.3168/jds.2019-17561
Lemke, S. L., S. E. Ottinger, K. Mayura, C. L. Ake, K. Pimpukdee, N. Wang, and T. D. Phillips. 2001. Development of a multi-tiered approach to the in vitro pre-screening of clay-based enterosorbents. Anim. Feed Sci. Technol. 93:17-29. doi:10.1016/S0377-8401(01)00272-3
Maki, C. R., S. Haney, M. Wang, S. H. Ward, B. J. Rude, R. H. Bailey, R. B. Harvey, and T. D. Phillips. 2017. Calcium montmorillonite clay for the reduction of aflatoxin residues in milk and dairy products. J. Dairy Vet. Sci. 2:1-8. https://doi.org/10.19080/JDVS.2017.02.555587
Moretti, A., M. Pascale, and A. F. Logrieco. 2019. Mycotoxin risks under a climate change scenario in Europe. Trends in Food Sci. Technol. 84: 38-40. doi:10.1016/j.tifs.2018.03.008
Mortland, M. M., and J. G. Lawless. 1983. Smectite interaction with riboflavin. Clays. Clay Miner. 31:435-439. doi:10.1346/CCMN.1983.0310604
Pimpukdee, K., L. F. Kubena, C. A. Bailey, H. J. Huebner, E. Afriyie-Gyawu, and T. D. Phillips. 2004. Aflatoxin-induced toxicity and depletion of hepatic vitamin a in young broiler chicks: protection of chicks in the presence of low levels of NovaSil PLUS in the diet. Poult. Sci. 83:737-744. doi:10.1093/ps/83.5.737
Ralla, K., U. Sohling, D. Riechers, C. Kasper, F. Ruf, and T. Scheper. 2010. Adsorption and separation of proteins by a smectite clay mineral. Bioprocess Biosyst. Eng. 33:847-861. doi:10.1007/s00449-010-0408-8
Sulzberger, S. A., S. Melnichenko, and F. Cardoso. 2017. Effects of clay after an aflatoxin challenge on aflatoxin clearance, milk production, and metabolism of Holstein cows. J. Dairy Sci. 100:1856-1869. https://doi.org/10.3168/jds.2016-11612
Tomasevic-Canovic, M., A. Dakovic, and V. Markovic. 2001. The effect of exchangeable cations in clinoptilolite and montmorillonite on the adsorption of aflatoxin B1. J. Serb. Chem. Soc. 66:555-561. doi:10.2298/jsc0108555t
Vekiru, E., S. Fruhauf, M. Sahin, F. Ottner, G. Schatzmayr, and R. Krska. 2007. Investigation of various adsorbents for their ability to bind aflatoxin B1. Mycotoxin. Res. 23:27-33. doi:10.1007/BF02946021