Deepthi B V and M Y Sreenivasa
Department of Studies in Microbiology, University of Mysore, India.
Email: [email protected]; [email protected]
Fumonisins are mycotoxins majorly produced by Fusarium verticillioides and Fusarium proliferatum species and are predominantly associated with maize and maize-based poultry and animal feeds (Dass et al., 2008; Sreenivasa et al., 2011; Deepa et al., 2016).
Cereal grains and their by-products, being major ingredients of feeds, represent an excellent substrate for the growth and reproduction of fungi and have serious consequences on the safety of foods and feeds.
In a mycological study carried out by our laboratory, 45.37% of feed mixtures collected all over Karnataka (India) showed contamination with fumonisin-producing Fusarium species (Figure 1).
⇰ Furthermore, the study revealed a frequency of 25% and 64.28% of Fusarium contamination in animal and poultry feeds, respectively.
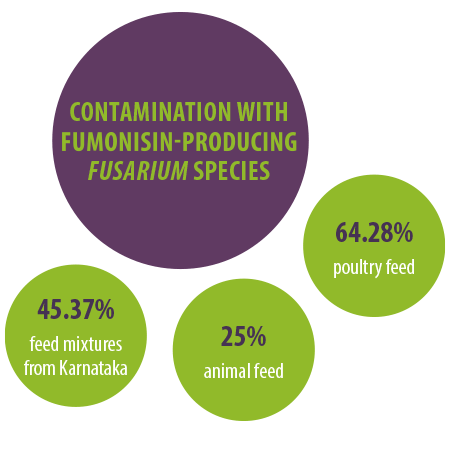
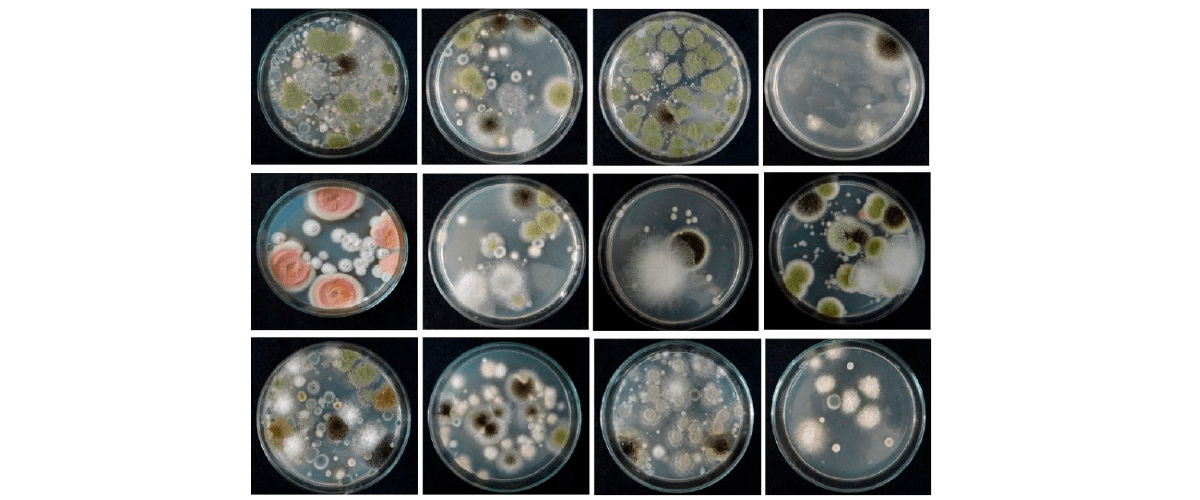
Figure 1. Fusarium and other mycotoxigenic fungal species isolated from animal and poultry feed mixtures.
On the other hand, other fungal genera such as Aspergillus flavus, A. columnaris, A. candidus, A. niger, A. parasiticus, Penicillium, Cladosporium, Rhizopus, Helminthosporium, Mucor, sterile hyphae, etc. showed a frequency of 50% and 82.1% in animal and poultry feeds, respectively (Figure 2).
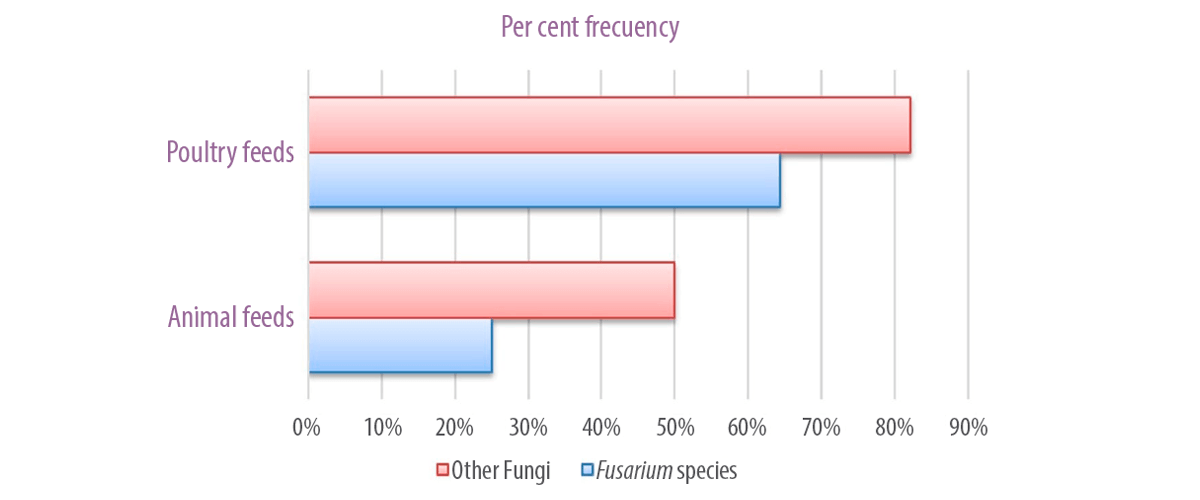
Figure 2. Per cent frequency of animal and poultry feeds showing contamination of Fusarium and other fungi.