Antonio Gallo
Associate Professor, Department of animal science, food and nutrition, Università Cattolica del Sacro Cuore, Italy
Mycotoxins are molecules produced by filamentous fungi. In particular, they are secondary metabolites of moulds belonging to several genera.
The term “secondary metabolites” refers to the fact that no obvious role is attributed to the mycotoxin for the growth or reproduction of the organisms that produce them.
Mycotoxins are mainly produced as a result of the interaction between the fungus and the surrounding environment.
Without fungal growth, there are no mycotoxins. However, the presence of moulds does not assure the presence of mycotoxins.
⇰ Only specific strains can lead to the production of mycotoxins and the productions of these substances require specific environmental conditions.
Mycotoxins have low molecular weight and they are very stable, eliciting a toxic response, both in humans and other vertebrate animals (Gallo et al., 2015).
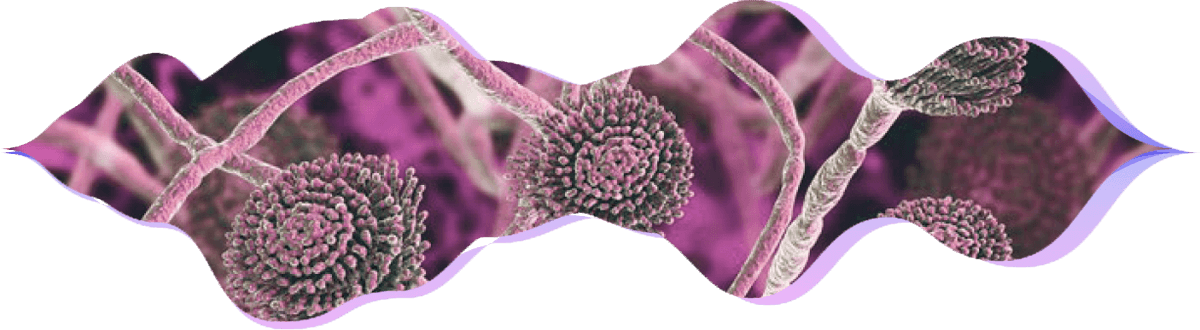
The main mycotoxigenic fungi belong to the genera Alternaria, Aspergillus, Fusarium, Monascus and Penicillium spp. but other genera, such as Chaetomium, Cladosporium, Claviceps, Diplodia, Myrothecium, Phoma, Phomopsis, Pithomyces, Trichoderma and Stachybotrys, also include mycotoxigenic species.

Figure 1. Main mycotoxigenic fungi. Alternaria, Aspergillus, Fusarium and Penicillium spp.
There are about 18,000 fungal secondary metabolites described in the AntiBase database, but only a limited number of those has received scientific interest from the ‘60s and onwards (Gallo et al., 2015).
The most studied mycotoxins are regulated mycotoxins are:
- ⇰ Aflatoxins (AF)
- ⇰ Citrinin
- ⇰ Trichothecenes, such as deoxynivalenol (DON)
- ⇰ Patulin
- ⇰ Ochratoxin A (OTA)
- ⇰ Fumonisins (FBs)
- ⇰ Zearalenone (ZEN)
- ⇰ Some major toxins of endophytic fungi: ergot toxins and ergotamine
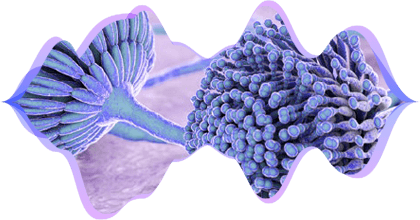
Mycotoxicosis are the syndromes resulting from ingestion, skin contact, or inhalation of these fungal metabolites by higher organisms like humans and livestock.
⇰ Mycotoxins can be teratogenic, mutagenic, and carcinogenic and they can cause various toxic effects, both in humans and in animals when ingested, inhaled or absorbed by skin contact.
The toxic effects of mycotoxins depend on the:
- ⇰ Type of toxin
- ⇰ Dose
- ⇰ Target organ
- ⇰ Sex, age and species
When livestock ingests mycotoxin-contaminated feed contaminated, the effects on their health may be acute or chronic.
Acute effects are obvious signs of illness or even death. However, the observation of acute manifestations of mycotoxicosis is rare under farm conditions.
Chronic effects are other less obvious disorders, such as feed refusal and reduction of ingestion, productivity, fertility and immunosuppression.
Acute and chronic effects cause severe economic losses due to:
- ⇰ Clinically ambiguous changes in animal growth
- ⇰ Reduced feed intake or feed rejection
- ⇰ Altered nutrient absorption and metabolism
- ⇰ Endocrine effects
- ⇰ Impact of immune status
(Fink-Gremmels, 2008)
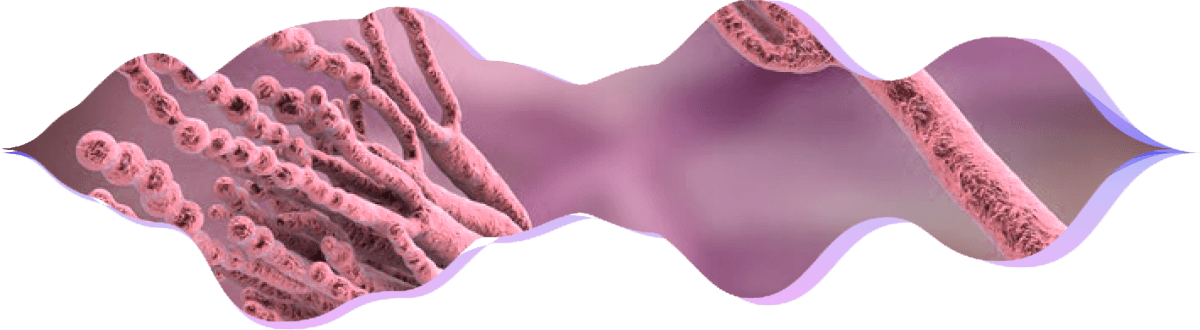
The effects of mycotoxins in ruminants are associated with a multitude of confounding effects and it is difficult to distinguish the effects due to ingestion of these toxins from other possible health problems. This is due to:
1. The effect of mycotoxins is related to several factors (diet, animal or environmental conditions).
2. The co-occurrence of multiple mycotoxins. The simultaneous presence of multiple mycotoxins will cause interactive effects, classified as additive, antagonistic, or synergistic (CAST, 2003).
The effects due to ingestion of multiple mycotoxins can therefore be similar, minor, or, in most cases, much greater than ingestion of the single mycotoxin.
3. Mycotoxins can be modified, primarily by the plant that can produce the hidden mycotoxins (mycotoxins conjugated to polar compounds such as glucose, malonic acid, and glutathione and this compound cannot be quantified by normal laboratory methods).
⇰ Modified forms of a mycotoxin likely exert the same toxicity as the original compound, being specific bonds hydrolysed in the digestive tract.
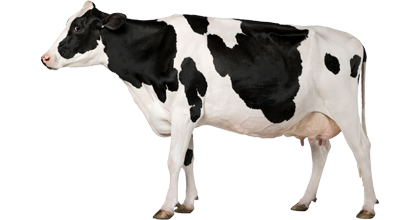
FUSARIUM MYCOTOXIN-INDUCED TOXICITY
Animals can develop numerous symptoms following the consumption of feed contaminated with mycotoxins produced by Fusarium spp., including gastrointestinal problems, soft stools, diarrhoea, immunosuppression, and a general decline in performance, likely due to reduced feed intake and increased susceptibility to disease (indirect cause) (Seeling et al., 2006).
The most relevant and with the highest incidence Fusarium mycotoxins are:
- ⇰ ZEN
- ⇰ DON
- ⇰ FB
- ⇰ T-2 toxin (T2) and HT-2 toxin (HT2)
- ⇰ Nivalenol (NIV)
- ⇰ Diacetoxyscirpenol (DAS)
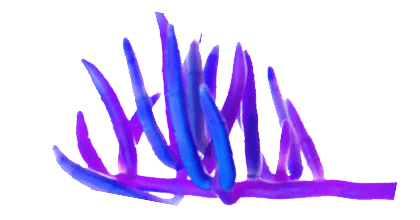
Fusarium mycotoxins and gut health
Fusarium mycotoxins can affect mucosal immunity that consists of an innate and adaptive immune system (Figure 2).
Figure 2. EThe effect of Fusarium mycotoxins on the intestinal epithelium. Fusarium mycotoxins alter different intestinal defense mechanisms, including epithelial integrity, cell proliferation, mucus layer, immunoglobulins (Ig) and cytokine (adapted from Antonnisen et al., 2014).

Fusarium mycotoxins are able to cross the intestinal epithelium and reach the systemic compartment, affecting the immune system.
⇰ These toxins can have immunostimulatory or immunosuppressive effects that depend on the age of the host, as well as the exposure dose and duration.
Fusarium mycotoxins and oxidative stress
Ruminants are a potential source of microbes or enzymes for mycotoxins biodegradation.
For example, mycotoxins are metabolized by cytochrome P450 enzymes to metaboliteguanineN7 adduct (Figure 3).
Figure 3. Mycotoxin metabolism in vertebrates (Adaptado de Omar HEM.,2013).
Cytotoxicity and ROS (Reactive Oxygen Species) generation are mechanisms of mycotoxin-mediated toxicity.
ROS are chemically reactive molecules containing oxygen formed as a natural by-product of the normal metabolism of oxygen and they have important roles in cell signalling and homeostasis.
In situations of environmental stress, ROS levels can increase dramatically as a result of oxidative stress.
⇰ Oxidative stress is present when the concentration of ROS generated exceeds the antioxidant capability of the cell and it results in various deleterious processes due to an imbalance between the excessive formation of ROS and limited antioxidant defences.
The cell can tolerate small and moderate amounts of oxidative stress by producing antioxidant molecules, such as vitamin A, C & E, and GSH, as well as activating enzymes (e.g., CAT, SOD, GPx, glutathione reductase [GR] and glutathione S-transferase [GST]) to counteract the excess oxidants.
⇰ One the other hand, lipoxygenases (LPO) may bring about protein damage and inactivation of membrane-bound enzymes through direct attack, either by free radicals or through chemical modification by its products.
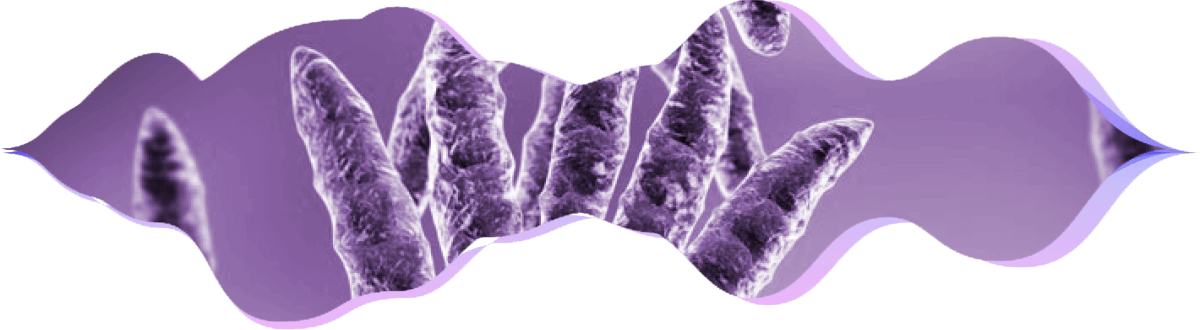
THE EFFECTS OF MYCOTOXINS ON RUMINANTS, WITH PARTICULAR ATTENTION TO PERFORMANCE AND DIGESTIBILITY
In 2015, Gallo et al. reviewed extensively all the present bibliography on mycotoxins, creating a summary able to collect all the tests conducted so far.
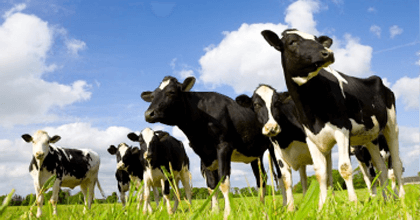

Table 1 shows the bibliographic upgrade from 2015 to 2021 of the tests conducted in vivo relative to the effects of Fusarium mycotoxins.
In general, responses related to the type of the mycotoxins were seen on:
- ⇰ Feeding behaviour and digestibility of the diet
- ⇰ Physiological and immune state
- ⇰ Milk production and quality
- ⇰ Reproductive aspects
- ⇰ Changes in body weight
Table 1. Effects of Fusarium mycotoxins ingestion in ruminants.
*Recalculated from data presented in each article AMH: Anti-Müllerian hormone; SCC: Somatic Cell Count; SA:SO ratio: Sphinganine/Sphingosine ratio; ADG: Average Daily Gain; CP: Crude Protein; ENN: Enniatins; MPA: Mycophenolic acid; ROQ-C: Roquefortine C; VFA: Volatil Fatty Acids
For a more intuitive representation, Figure 4 summarizes the effects of 21 trials, published in peer-reviewed journals, that were conducted to evaluate the effect of mycotoxins produced by Fusarium spp. (i.e., mainly DON, ZEN and FB).
Some trials were conducted on a single mycotoxin, while others evaluated the effect of different mycotoxins at the same time.
⇰ When mycotoxins with different effects were studied in the same trial, each effect was attributed only to the mycotoxin responsible and not to the whole complex of mycotoxins examined in the trial for data processing.
The data obtained was then relativized regarding the total number of tests conducted and examined for a particular mycotoxin, subsequently calculating how many tests showed a particular effect, which was finally expressed in terms of percentage incidence.
Figure 4 shows, in a very intuitive way, that:
⇰ ZEN is linked to reproductive effects ⇰ FBs are linked to effects on the digestibility of the diet, feeding behaviour, immune physiological state and body weight ⇰ DON has a wide spectrum of action and involves all the spheres affected by FB, as well as the production and quality of milk |
Figure 4. The effects of 21 trials conducted evaluating the effect of mycotoxins DON, ZEN and FB tested both alone and in combination with each other.
Bibliography: Duringer J.M. et al; 2020; World Myco. J.- Roberts H. L. et al.; 2021; Toxins- Gallo A. et al; 2020; J. Dairy Sci.- Danicke S. et al.; 2016; Arch. Anim. Nutrit. – Jovaisiene J. et al.; 2016; Pol. Jour. Vet. S. – Kinoshita A. et al.; 2015; J. of Phys. and Anim. Nutr.- Jennings J.S. et al.; 2020; J. Anim. Sci. – Fushimi Y. et al.; 2015; Reprod Dom Anim. – Almeida Silva L. et al.; 2021; Reprod. Dom. Anim. – McKay et al., (2019); Anim. Feed Sci. Technol. – Hildebrand B. et al; 2012; J. Anim. Physiol. Anim. Nutr. – Keese, C.; 2008; Arch. Anim. Nutr. – Keese, C.; 2008; Arch. Anim. Nutr. – Korosteleva, S.N.; 2007; J. Dairy Sci. – Ingalls, J.R.; 1996; Anim. Feed Sci. Technol. – Weaver, G.A.; 1986; Am. J. Vet. Res. – Coppock, R.W.; 1990; Vet. Hum. Toxicol. – Baker, D.C.; 1999; J. Vet. Diagn. Investig.- Osweiler, G.D.; 1993; J. Anim. Sci. – Mathur, S.; 2001; Toxicol. Sci.- Weaver, G.A.; 1986; Am. J. Vet. Res.
In 2008, Fink-Gremmels was able to demonstrate that ruminants are less susceptible than monogastric animals if they are fed mycotoxin-contaminated feed.
The rumen represents an active defense as it has a binding action towards mycotoxins (they are retained through fibre, yeast walls, bacterial walls) or it has mechanisms for deactivation/degradation of these secondary metabolites (due to protozoa or bacteria that are present in the rumen).
Unlike monogastric animals, which receive a highly standardized diet rich in starchy products and poor in fibre, ruminants receive an extremely varied diet that includes silage obtained from various matrix (corn, barley, wheat, sorghum), hay, haylage, high moisture corn, by-products, seeds and other concentrates.
Due to the need to feed ruminants with feedstuffs that are not competitive with human food, and to be able to react to high raw material prices, ruminant diet formulation is changing and the inclusion of co- and by-products is increasing.
![]() |
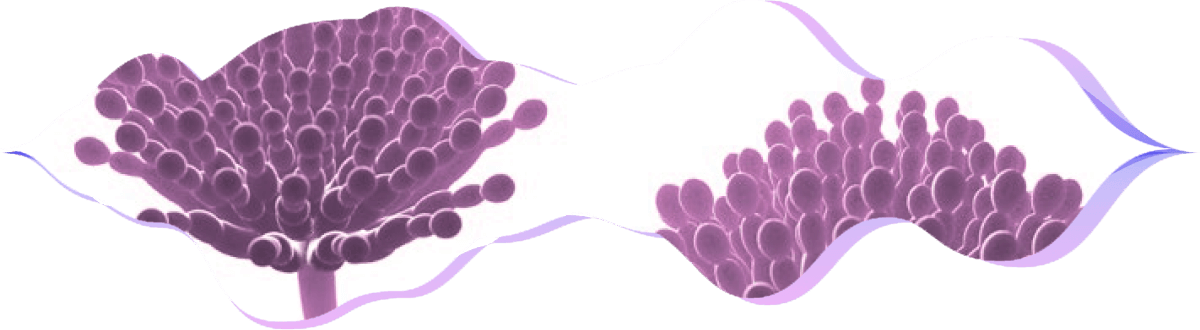
A trial recently conducted by Gallo et al. (2020) studied the effects of regular levels (levels naturally found in food) of contamination of DON and FBs in total mixed ration (TMR) on performance, digestibility of the diet, milk quality and plasma liver enzymes in dairy cows.
The experiment included a:
- ⇰ Control diet (CTR)
- ⇰ Diet contaminated with mycotoxins (MTX)
- ⇰ Diet contaminated with mycotoxins added with a mycotoxin deactivating product (MDP)
Body condition and feed intake
No changes were found for body condition score (BCS), dry matter intake (DMI) and body weight (BW) during the trial when lactating dairy cows were fed with the three experimental diets (Figure 5).
Figure 5. Effects of regular levels of mycotoxin contamination on TMR intake and body weight.
Milk yield and quality
Comparison of milk production showed that the MTX diet caused a reduction in milk yield of 1.34 kg/cow/day compared to the CTR diet. The MDP diet caused a milk yield reduction of 1.14 kg/cow/ day, when compared to the CTR diet (Figure 6).
|
Figure 6. Effects of regular levels of mycotoxin contamination on milk yield.
Regarding milk quality parameters, curd firmness and firming time were negatively affected by the MTX diet compared to the other 2 diets (CTR and MDP diet; Figure 7).
⇰ Therefore, the deactivating product for mycotoxins had positive practical results, being able to counteract the negative effects of mycotoxins in rennet coagulation properties of milk.
Figure 7. Effects of regular levels of mycotoxin contamination on clotting time, curd firmness and curd firming time.
Immune status
Compared to the CTR diet, the MTX diet resulted in slightly lower expression of genes associated with immune and inflammatory functions, indicating that Fusarium mycotoxins had an immunosuppressive effect.
The trial revealed that diets contaminated with regular levels of Fusarium mycotoxins adversely affected lactating dairy cow performance, milk quality, digestibility of the diet, metabolic profile and immunity, and that supplementation with a commercial mycotoxin deactivator product counteracted most of these negative effects, but not milk yield. |
In 2021, Gallo et al. conducted a new study on the effects of Fusarium mycotoxins on the in vitro fermentation potential of ruminal fluid sampled from lactating dairy cows ingesting contaminated diets with regular levels of these mycotoxins.
Higher final volumes or gas production rates were observed over time for ruminal MDP inoculum than for MTX inoculum (Figure 8), but treatment-feed interaction was observed.
Figure 8. Gas production (ml/g OM) in relation to the three different treatments.
Concentrations of volatile fatty acids (VFA) were not different between treatments, with the exception of a slight increase in acetic acid in CTR compared to MTX (Figure 9).
Figure 9. VFA proportion in rumen inoculum after 48h.
Gallo et al., in another article published in 2021, characterized silage samples in terms of the presence of chemicals, bacterial community and concentrations of various fungal metabolites.
The silages were grouped into five groups, based on the mycotoxins detected and the study showed that corn silage can be contaminated by a high number of regulated and emerging mycotoxins.
This is consistent with reports by Santos et al. (2014) that observed severe BCS losses, poor stool consistency, rigid gait and signs of laminitis without clear signs of disease associated with irregular increases in somatic cell count and low milk production, thereby excluding infectious or metabolic diseases.
⇰ However, visual analysis of silage showed aerobic instability and mould growth throughout the silo, which was again confirmed by TMR analysis showing multiple mycotoxin contamination.
The current and next areas of research that are being pursued are:
- ⇰ The creation and improvement of the quality index of fermentation of corn silage (as reported by Gallo et al, 2016)
- ⇰ The risk index of mycotoxins in corn silage
- ⇰ NIR calibrations for the characterization of mycotoxins in corn silage
The application of NIR to clustering maize silages for mycotoxin content has yielded extremely positive results that could allow to determine very quickly whether a sample is contaminated with mycotoxins or not (Figure 10).
Figure 10. NIR application for clustering corn silage for mycotoxin content (Discriminant Random Forests analysis). PhD project of Ghilardelli Francesca.
REFERENCES
Gallo, A., Giuberti, G., Frisvad, J. C., Bertuzzi, T., & Nielsen, K. F. (2015). Review on mycotoxin issues in ruminants: Occurrence in forages, effects of mycotoxin ingestion on health status and animal performance and practical strategies to counteract their negative effects. Toxins, 7(8), 3057-3111.
Seeling, K., Lebzien, P., Dänicke, S., Spilke, J., Südekum, K. H., & Flachowsky, G. (2006). Effects of level of feed intake and Fusarium toxin‐contaminated wheat on rumen fermentation as well as on blood and milk parameters in cows. Journal of Animal Physiology and Animal Nutrition, 90(3‐4), 103-115.
Antonissen, G., Martel, A., Pasmans, F., Ducatelle, R., Verbrugghe, E., Vandenbroucke, V., Shaoji, L., Haesebrouck F., Van Immerseel, F. & Croubels, S. (2014). The impact of Fusarium mycotoxins on human and animal host susceptibility to infectious diseases. Toxins, 6(2), 430-452.Omar, H. E. M. (2013). Mycotoxins-induced oxidative stress and disease. Mycotoxin and Food Safety in Developing Countries. Rijeka, Croatia: InTech, 63-92.
Omar, H. E. M. (2013). Mycotoxins-induced oxidative stress and disease. Mycotoxin and Food Safety in Developing Countries. Rijeka, Croatia: InTech, 63-92.
Fink-Gremmels, J. (2008). The role of mycotoxins in the health and performance of dairy cows. The Veterinary Journal, 176(1), 84-92.
Gallo, A., Minuti, A., Bani, P., Bertuzzi, T., Cappelli, F. P., Doupovec, B., J. Fass, D. Schatzmayr, & Trevisi, E. (2020). A mycotoxin-deactivating feed additive counteracts the adverse effects of regular levels of Fusarium mycotoxins in dairy cows. Journal of dairy science, 103(12), 11314-11331.
Gallo, A., Ghilardelli, F., Doupovec, B., Faas, J., Schatzmayr, D., & Masoero, F. (2021). Kinetics of gas production in the presence of Fusarium mycotoxins in rumen fluid of lactating dairy cows. JDS Communications, 2(5), 243-247.
Gallo, A., Ghilardelli, F., Atzori, A. S., Zara, S., Novak, B., Faas, J., & Fancello, F. (2021). Co-occurrence of regulated and emerging mycotoxins in corn silage: Relationships with fermentation quality and bacterial communities. Toxins, 13(3), 232.
Santos, R. R., & Fink-Gremmels, J. (2014). Mycotoxin syndrome in dairy cattle: Characterisation and intervention results. World Mycotoxin Journal, 7(3), 357-366.
Gallo, A., Giuberti, G., Bruschi, S., Fortunati, P., & Masoero, F. (2016). Use of principal factor analysis to generate a corn silage fermentative quality index to rank well‐or poorly preserved forages. Journal of the Science of Food and Agriculture, 96(5), 1686-1696.