Assistant Prof. Panagiotis Tassis
Assistant Professor of Swine Medicine and Reproduction, Clinic of Farm Animals, School of Veterinary Medicine, Aristotle University of Thessaloníki, Greece
Pig farm vaccination programs are a major preventive tool for a wide range of diseases and syndromes affecting swine. They have colossal importance in terms of herd health and productivity, as well as from a financial standpoint.
Therefore, proper vaccine selection and proper implementation are the basis for the construction of a herd immune status that will counteract antigenic pressure during different productive stages.
As already discussed in the respective literature, mycotoxins seem to play an important role in disrupting this major preventive health tool1.
In our previous technical article regarding the effects of major mycotoxins on the immune system of swine and cellular and molecular mechanisms involved, it had been reported that the health and economic impact of mycotoxins on the immune defense system of pigs is significant.
Three major outcomes of these effects on the swine immune system, herd health and productivity have been described2:
- ⇰ Increased susceptibility to infectious diseases
- ⇰ Reactivation of chronic infections
- ⇰ Decreased vaccination efficacy
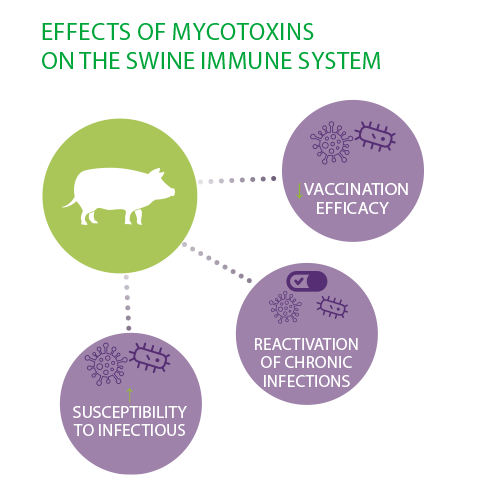
The current knowledge on the effects of aflatoxins (AFs), fumonisins (FBs and mainly FB1) deoxynivalenol (DON), zearalenone (ZEN), ochratoxin A (OTA) and T-2 toxin, on the immune response after sensitization or vaccination in swine will be presented.
vaccines against swine pathogens.
Swine vaccines and vaccinal immunity
The use of veterinary vaccines in swine production is a disease prevention tool that has been used by swine farmers worldwide in a variety of production systems.
It is still implemented in every conventional pig production system. In the past few decades, facts in the field of novel vaccine production have changed rapidly.
The scientific field of swine vaccine development is a rapidly evolving research and innovation field.
Major swine diseases that can be prevented or controlled at field level with the use of commercially available vaccines, as part of a veterinary health management programme, include:
- ✔ Porcine Reproductive and Respiratory Syndrome (PRRS)
- ✔ Porcine Circovirus 2-associated diseases (PCV2-AD)
- ✔ Aujeszky’s disease (PRV)
- ✔ Parvovirus infection (PPV)
- ✔ Swine influenza (SIV)
- ✔ Enzootic pneumonia (Mycoplasma hyopneumoniae)
- ✔ Pleuropneumonia (App – Actinobacillus Pleuropneumoniae)
- ✔ Glasser’s disease (Haemophilus parasuis)
- ✔ Atrophic Rhinitis (Pasteurella multocida ± Bordetella bronchiseptica)
- ✔ Erysipelas (Erysipelothrix rhusiopathiae)
- ✔ Leptospirosis (Leptospira spp.)
- ✔ Escherichia coli infections
- ✔ Clostridium spp. infections
- ✔ Ileitis (Lawsonia intracellularis)
- ✔ Classical Swine Fever
- ✔ Salmonella spp. and others

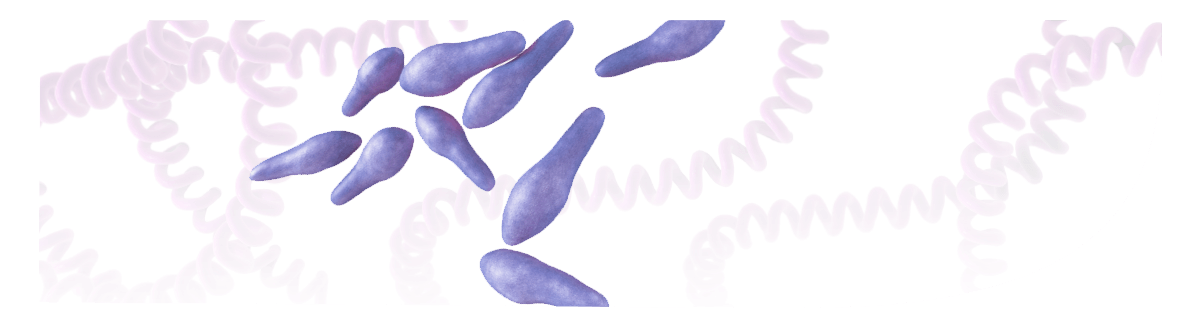
Apart from typical intramuscular vaccination against one pathogen, innovations of vaccine technology in the past decades have resulted in the production of intradermal vaccines, intranasal vaccines, as well as vaccines against more than one pathogen, reaching up to three swine pathogens in one vaccine up today.
Swine vaccines are usually either “dead” (inactivated) or “live” (attenuated) and can be used in different production stages in the breeding stock and/or in suckling, weaned or growing pigs, depending on the vaccine, its pathogenic target and suggested administration programme.
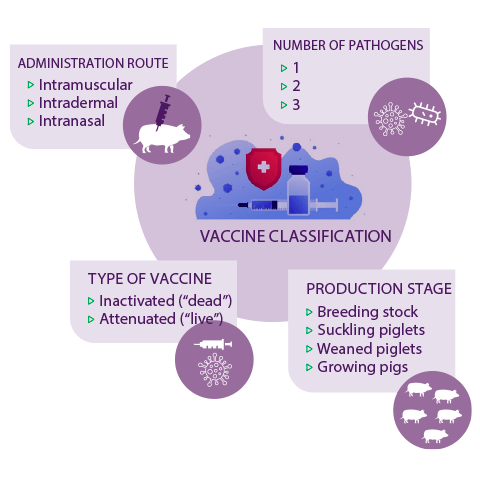