Abdelhacib Kihal
DVM, MSc, PhD in Animal Production
Department of Animal and Food Science, Universitat Autònoma de Barcelona, Spain
Mycotoxicosis occurs as a consequence of the consumption of food contaminated with mycotoxins.
The induced effects are subclinical and difficult to diagnose, but cause significant production losses.
More than 400 mycotoxins have been identified, six of them classified as highly toxic and frequent in animal feed:
- ⇰ Aflatoxins
- ⇰ Ochratoxin A
- ⇰ Fumonisins
- ⇰ Deoxynivalenol
- ⇰ Zearalenone
- ⇰ T-2 toxin
(Krska et al., 2016)
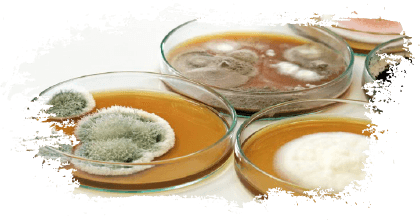

Among the variety of mycotoxin control methods (Figure 1), mycotoxin adsorbents (ADS) are an effective strategy to sequester mycotoxins in their matrix and prevent their absorption through the gastrointestinal tract (GI tract) of animals (Čolović et al., 2019).
⇰ As a result, the mycotoxin-ADS complex passes through the GI tract of the animals and is excreted in the feces (Gimeno y Martins, 2007).
The European Food Safety Authority (EFSA, 2011) requires the efficacy of a mycotoxin adsorbent should be evaluated by one in vitro test and two in vivo tests.
These tests must demonstrate a high affinity and speed for adsorbing mycotoxins at a low inclusion rate of the mycotoxin adsorbent in the diet that will allow a high degree of adsorption, but without interfering with other nutrients.
Figure 1. Different mycotoxin control methods.
Classification of mycotoxin adsorbents
Mycotoxin adsorbents are classified, according to their nature, into two main groups:
- ⇰ Inorganic adsorbents
- ⇰ Organic adsorbents
Inorganic adsorbents
SILICATES
Silicates represent the most important group of inorganic adsorbents.
⇰ Tetrahedral sheets are the basis of these adsorbents in which different silicate subgroups are formed in combination with different mineral ions in two- or threedimensional structures (Figure 2).
Figure 2. Molecular structure of octahedral and tetrahedral tectosilicate sheets, and an illustration of the contribution of ions to the adsorption mechanism of mycotoxins. (Kihal et al., 2022; created with BioRender.com).
PHYLOSILICATES
Phyllosilicates are the first group of silicates. They are formed by stacking tetrahedral and octahedral sheets in two main combinations:
- ⇰ A 1:1 tetrahedral and octahedral sheet
- ⇰ Two tetrahedral sheets and one octahedral sheet in the middle 2:1
(Figure 2, Murray, 2007)
The interlaminar space between the sheets and the presence of cations with different charges are responsible for the adsorption capacity of mycotoxins.
TECTOSILICATES
The second group of silicates is that of the tectosilicates, which are formed by the assembly of multiple tetrahedral structures in a three-dimensional form.
⇰ This arrangement generates different pores with the same dimensions, resulting in a ring or cage structure. The pores formed by the three-dimensional structure are the basis of the adsorption capacity where the potassium and calcium cations inside interact with the mycotoxins (Nadziakiewicza et al., 2019).
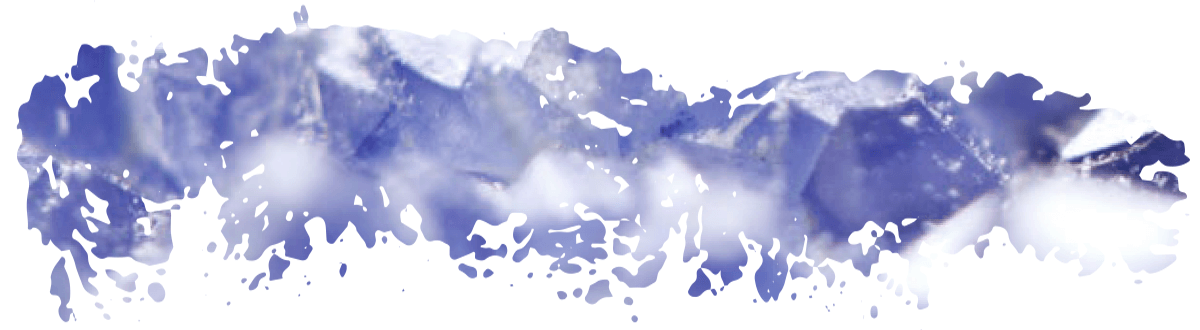
ACTIVATED CARBON
Apart from silicates, activated carbon is also classified as an inorganic adsorbent.
It is a non-soluble powder formed by the carbonization of organic compounds containing carbon through a pyrolysis heating process at temperatures up to 2.000ºC (Galvano et al., 1996).
⇰ The resulting powder requires an activation process necessary to acquire a higher adsorption capacity through chemical or physical processes that allow the formation of many highly porous structures (Figura 3).
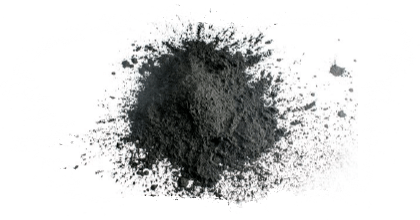
Figure 3. Microscopic view of activated carbon micropores and how mycotoxins and nutrients are adsorbed within the pores depending on their molecular size (Kihal et al., 2022).
Organic adsorbents
YEAST CELL WALLS
The most important adsorbents of this group are yeast cell walls (YCW), with their β-D-glucans being the main factor that determines their adsorption capacity.
⇰ This capacity increases as the proportion of β-D-glucans present in the yeast strain is increased (Yiannikouris et al., 2004).
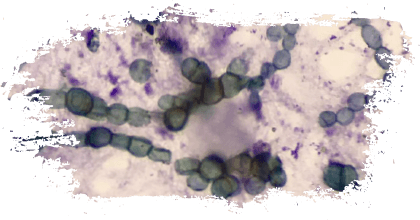
Figure 4. Composition of the different layers of the yeast cell wall (Kihal et al., 2022; created with BioRender).
Adsorbent effectiveness
Mycotoxin adsorption capacity is determined by factors related to the adsorbent, but also to the physicochemical characteristics of the mycotoxins.
Mycotoxin adsorbent properties
SILICATES
The cation exchange capacity and the total net surface charges determine the ability of silicate adsorbents to adsorb mycotoxins. However, this exchange capacity depends, to a large extent, on the adsorbent’s pH.
Each adsorbent has its own pH, pH of point zero charge, where the adsorbent surface has equal positive and negative charges.
⇰ If the pH of the medium is lower than that of the adsorbent, hydrogen ions bind to the adsorbent, which loses its charge.
⇰ If the pH of the medium is higher than that of the adsorbent, the adsorbent will release hydrogen ions and expose negative charges, increasing the capacity to attract cations responsible for the interaction with mycotoxins.
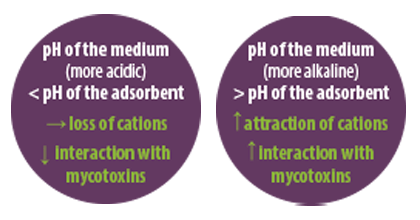
In addition to the external cation bond, the interlaminar space in the mycotoxin adsorbent also plays an important role, so, the larger it is, the greater will be the adsorption capacity of the mycotoxin adsorbent.

ACTIVATED CARBON
For activated carbon, the adsorption mechanism depends on factors such as pore size and surface area (Goto et al., 2015).
Unlike clay minerals, the adsorption mechanism of activated carbon is due to hydrophobic interactions and pi bonds.
In addition, pore size and pore distribution within the activated carbon are also limiting factors that determine the adsorption efficiency.
YEAST CELL WALLS
The adsorption mechanism of yeast cell walls is mainly due to β-glucans.
The links involved in this interaction are:
- ⇰ Van der Walls bonds between the aromatic cycle of the mycotoxins and the β-D-glucopyranose ring of the YCW.
- ⇰ Hydrogen bonds between the hydroxyl, ketone, and lactone groups of mycotoxins and the hydroxyl group of the glucose units of β-D-glucans in the YCWs.
(Jouany et al., 2005)
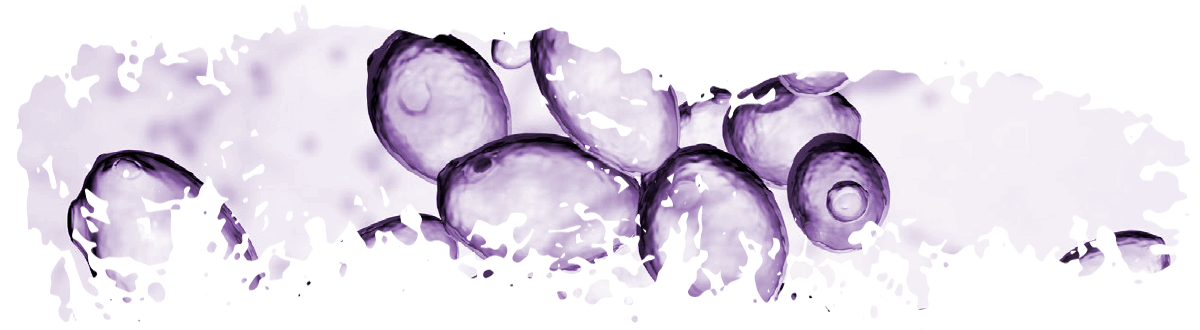
Mycotoxin properties
The physicochemical characteristics of mycotoxins also affect the adsorption capacity of adsorbents (Galvano et al., 1997).
Mycotoxins can be classified according to their:
- ⇰ Polarity
- ⇰ Solubility
- ⇰ Chemical structure
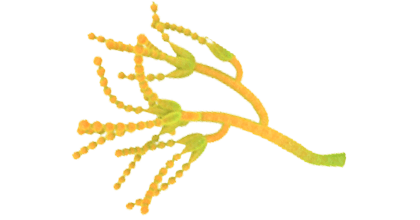
POLARITY
The polarity of mycotoxins reflects the charge arrangement within the molecule and can be classified as polar or non-polar.
SOLUBILITY
The solubility of mycotoxins in the medium is important for their adsorption.
⇰ Most of them are soluble in different organic solvents. However, their solubility in water depends on their polarity, with the most polar mycotoxins being the most soluble.
CHEMICAL STRUCTURE
The chemical structure, size and shape of the molecule also affect its adsorption. Mycotoxin adsorption has a direct relationship with the size of the molecules.
⇰ In this sense, mycotoxins with a small size, such as aflatoxins, are adsorbed better than mycotoxins with a larger size, such as fumonisins.
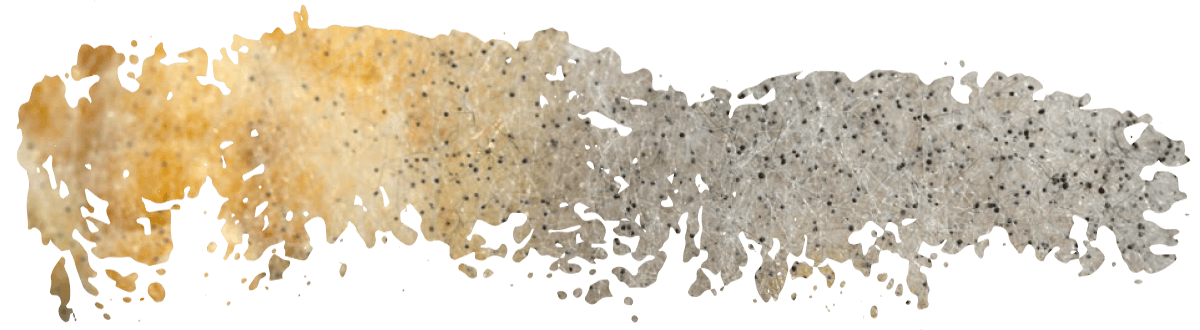
Methods for determining the adsorption capacity of mycotoxin adsorbents
The adsorption capacity of mycotoxin adsorbents is mainly determined by in vitro tests.
These tests have the advantage of being simple, fast and inexpensive.
Generally, these tests consist of incubating the mycotoxins with the adsorbents at different doses in a medium that simulates intestinal digestion under certain pH, timing, and temperature conditions.
There are other more complex models, but less frequently applied, such as the dynamic gastrointestinal model that mimics the conditions of the stomach and small intestine in a continuous way, or ex vivo models based on live cell enrichment.
⇰ These adsorption tests have many limitations that result in high variability between studies.
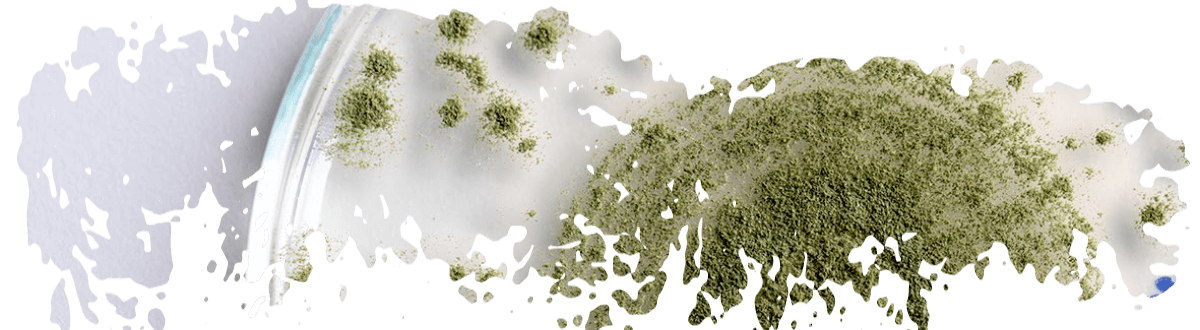
REFERENCES
Čolović, R., N. Puvaca, F. Cheli, G. Avantaggiato, D. Greco, O. Duragic, and L. Pinotti. 2019. Decontamination of mycotoxin-contaminated feedstuffs and compound feed. Toxins. 11:617. doi:10.3390/toxins11110617
European Food Safety Authority (EFSA), 2011. Opinion of the Scientific Panel on Additives and Products or Substances used in Animal Feed (FEEDAP Panel) on the safety and efficacy of bentonite (dioctahedral montmorillonite) as feed additive for all species. EFSA J. 9:2007. doi:10.2903/j.efsa.2014.3694
Galvano, F., A. Pietri, B. Fallico, T. Bertuzzi, S. Scire, M. Galvano, and R. Maggiore. 1996. Activated carbons: in vitro affinity for aflatoxin B1 and relation of adsorption ability to physicochemical parameters. J. Food Protec. 59:545-550. doi:10.4315/0362-028X-59.5.545
Galvano, F., A. Pietri, T. Bertuzzi, M. Bognanno, L. Chies, A. Angelis, and M. Galvano. 1997. Activated carbons: in vitro affinity for fumonisin B1 and relation of adsorption ability to physicochemical parameters. J. Food Protec. 60:985-991. doi:10.4315/0362-028X-60.8.985
Gimeno, A., and M. L. Martins. 2007. Micotoxinas y micotoxicosis en animales y humanos. Special nutrients. 1st edition. Douglas road, Miami, FL. USA. pp, 128.
Goto, T., Y. Amano, M. Machida, and F. Imazeki. 2015. Effect of polarity of activated carbon surface, solvent and adsorbate on adsorption of aromatic compounds from liquid phase. Chem. Pharm. Bull. 63:726-730. doi:10.1248/cpb.c15-00039
Gruber-Dorninger, C., T. Jenkins, and G. Schatzmayr. 2019. Global mycotoxin occurrence in feed: a ten-year survey. Toxins. 11:375. doi:10.3390/toxins11070375
Jouany, J. P., A. Yiannikouris, and G. Bertin. 2005. How yeast cell wall components can alleviate mycotoxicosis in animal production and improve the safety of edible animal products. J. Anim. Sci. 14:171-190. doi:10.22358/jafs/70361/2005
Kihal, A., M. E. Rodríguez-Prado, and S. Calsamiglia. 2022. The efficacy of mycotoxin binders to control mycotoxins in feeds and the potential risk of interactions with nutrient: a review. J. Anim. Sci. 100:1-14. doi:10.1093/jas/skac328
Kogan, G., and A. Kocher. 2007. Role of yeast cell wall polysaccharides in pig nutrition and health protection. Livest. Sci. 109:161-165. doi:10.1016/j.livsci.2007.01.134
Krska, R., M. de Nijs, O. McNerney, M. Pichler, J. Gilbert, S. Edwards, M. Suman, N. Magan, V. Rossi, H. J. van der Fels-Klerx, F. Bagi, B. Poschmaier, M. Sulyok, F. Berthiller, and H. P. van Egmond. 2016. Safe food and feed through an integrated toolbox for mycotoxin management: the MyToolBox approach. World Mycotox. J. 9:487- 495. doi:10.3920/WMJ2016.2136
Murray, H. H. 2007. Developments in clay science. Occurrences, processing and applications of kaolins, bentonites, palygorskitesepiolite, and common clays. Vol 2, 1 180. Elsevier Science & Technology, UK.
Nadziakiewicza, M., S. Kehoe, and P. Micek. 2019. Physico-chemical properties of clay minerals and their use as a health promoting feed additive. Animals. 9:714. doi:10.3390/ani9100714
Yiannikouris, A., J. François, L. Poughon, C. G. Dussap, G. Bertin, G. Jeminet, and J. P. Jouany. 2004. Adsorption of zearalenone by β-D-Glucans in the Saccharomyces cerevisiae cell wall. J. Food Prot. 67:1195-1200. doi:10.4315/0362-028x-67.6.1195