Jog Raj* and Marko Vasiljevic
PATENT CO, DOO., Vlade Ćetkovića 1A, 24 211, Mišićevo, Serbia
*Corresponding author: [email protected]
Mycotoxins are secondary harmful mold metabolites that produce significant detrimental health effects in human beings and animals (Haschek et al., 2013).
They are low molecular weight compounds considered to be harmful even at low concentrations (Milićević et al., 2010).
Approximately 25% of the crops, including cereal grains and nuts, are often presumed to be contaminated with fungi (Pandya et al., 2016, Raj et al., 2022).
To understand in depth the effects of mycotoxins and, particularly, Fumonisins, in animals and the strategies available for their remediation, this article is structured as follows:
- ⇰ Occurrence of mycotoxins
- ⇰ The effects of mycotoxins on animals
- ⇰ The effects of fumonisins on animals
- ⇰ Remediation of fumonisins using Minazel Plus® in vitro and in vivo
- ⇰ Conclusions
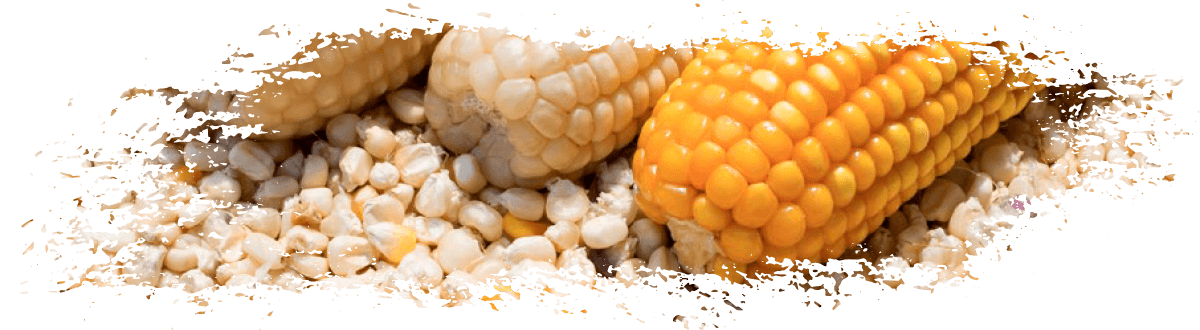
OCCURRENCE OF MYCOTOXINS
In 2022, PATENT CO DOO conducted a survey of harvested corn from 15 countries (Argentina, Bosnia & Herzegovina, Brazil, Chile, Colombia, Croatia, Ecuador, Guatemala, Italy, Mexico, Philippines, Peru, Serbia, South Africa, and Taiwan).
⇰ 96% of the samples were contaminated with one or more than one mycotoxin.
Among these 96% samples, 4% had 1 type of mycotoxin, 4% had 2 types of mycotoxins, 7% had 3 types of mycotoxins, and 81% had more than 3 types of mycotoxins present when analysed through LC-MS/MS (Figure 1).
Figure 1. Number of mycotoxins detected in corn samples from 15 countries in 2022 harvest.
For more information, read “Higher prevalence of Fumonisins and Fusaric acid in 2022 harvested corn”
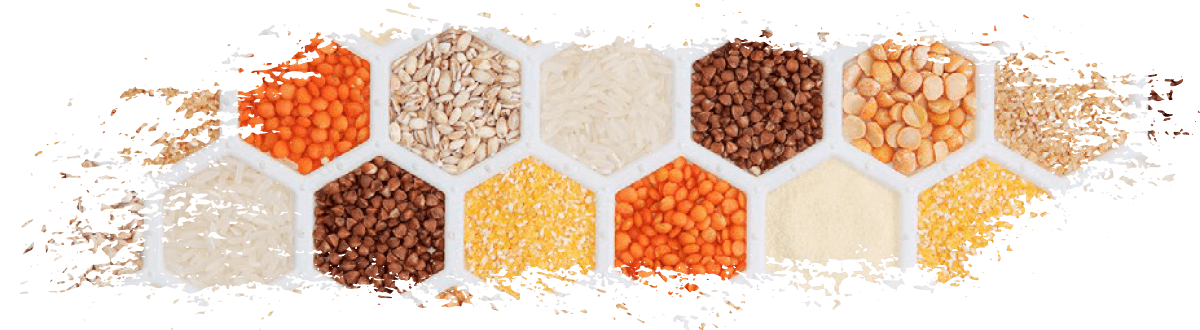
THE EFFECTS OF MYCOTOXINS ON ANIMALS
Among various mycotoxins, the most frequently encountered harmful mycotoxins in food stuff and feed are:
- ⇰ Aflatoxin B1 (AFB1)
- ⇰ Ochratoxin A (OTA)
- ⇰ Trichothecenes
- ⇰ Deoxynivalenol (DON)
- ⇰ HT-2 toxin
- ⇰ T-2 toxin
- ⇰ Fumonisin B1 (FB1)
- ⇰ Citrinin (CTN)
- ⇰ Zearalenone (ZEN)
- ⇰ Ergot Alkaloids
A predominately marked distribution of Fumonisins, Aflatoxins, Zearalenone and Deoxynivalenol is documented globally (Binder, 2007).
Cereal crops may be contaminated in the field itself or during harvesting, transport, processing, or storage practices (Coffee, 2006; Khazaeli et al., 2014).
The rate of contamination of crops with fungi is more frequently triggered by the rainy season (Pleadin et al., 2013, 2015, 2019).
Several known factors facilitate the production of mycotoxins in contaminated products, including:
- ⇰ Moisture content (20-25%)
- ⇰ Environmental temperature (22-30ºC)
- ⇰ Composition of food items
- ⇰ Relative air humidity (70-90%)
- ⇰ Physical damage to cereals by pests
- ⇰ pH
- ⇰ The presence of mold spores
(Pleadin et al., 2015)
Common routes of entry of mycotoxins to the body
The main route is direct consumption of contaminated products of plant origin (cereals, nuts, bread, etc.) and products obtained from animals (meat and meat products, milk, offal’s, fermented sausages, etc.) (Carvet et al., 2006; Pleadin et al., 2015).
Dermal contact and inhalation are not very common routes but can act as a potential mode of entry into the body (Creppy, 2002).
Toxic effects of mycotoxins
The harmful effects of mycotoxins depend on:
- ⇰ The type of mycotoxin
- ⇰ The dose introduced into the body
- ⇰ The duration of exposure to the mycotoxins
(Creppy, 2002; Speijers, 2004)
Figure 2. Classification of mycotoxins based on clinical manifestations.
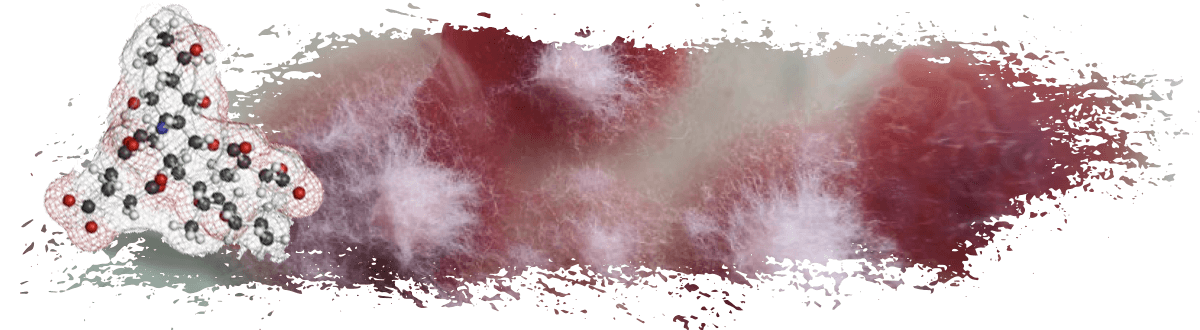
THE EFFECTS OF MYCOTOXINS ON ANIMALS
The summary of the effects of mycotoxins on animals is shown in Table 1.
Table 1. Summary of the main mycotoxins and their toxic effects.
THE EFFECTS OF FUMONISINS ON ANIMALS
Fumonisins are produced by fungal species such as Fusarium verticillioides and F. proliferatum.
These molds are frequently spotted on maize plants giving them a whitish appearance.
The most common forms of Fumonisins include A and B (B1, B2, B3, and hydrolyzed B1), Fumonisin B1 being the most common and potent one (Voss et al., 2014).
The production of this mycotoxin is promoted when the moisture content is <19%.
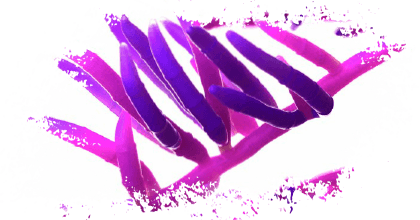
Fumonisin toxicity was first reported in 1980 as a cause of equine encephalomalacia (ELM) and porcine pulmonary oedema (PPE) in the United States, and esophageal cancer in Africa.
⇰ Fumonisins are also reported to cause leukoencephalomalacia in horses, hepatocellular carcinoma in rats and pulmonary oedema in association with hydrothorax in pigs.
The IARC has also documented the carcinogenic potential of Fumonisins in human beings (IARC, 2002).
The summary of the negative effects of Fumonisins is shown in Table 2.
Table 2. Clinical and pathoanatomical effects of Fumonisins.
Mechanisms of action of Fumonisins
Fumonisins compete with the ceramide synthase enzyme, causing oxidative stress along with endoplasmic reticulum stress, interfering with the modulation of autophagy and altering DNA methylation (Figure 3).
Figure 3. Mechanisms of inhibition of sphingolipid metabolism (Liu et al., 2019).
The effects of Fumonisins on horses
Fumonisins can be extremely harmful to horses. When present in corn or other grains, they can result in moldy corn poisoning or Leukoencephalomalacia.
⇰ The disease syndrome was named Leukoencephalomalacia due to the type (malacia = softening [due to necrosis]) and distribution (leuko = white matter) of the most prominent lesion in the brain.
Equines are the only species in which Fumonisins induce this kind of lesion.
Common symptoms are (Image 1):
- ⇰ Disorientation and walking aimlessly
- ⇰ Circling
- ⇰ Deranged behavior
- ⇰ Colic
- ⇰ Head pressing against solid objects
- ⇰ Blindness
- ⇰ Death in some cases
Image 1. Clinical presentation of horses affected by leukoencephalomalacia. (1-2) Horses presenting head pressing. (3-4) Horses present semi-comatose mental state and lateral recumbency (Source: Albertino et al., 2021).
The effects of Fumonisins in swine
Swine chronically intoxicated with FB1 and FB2, even at low doses, cause non-specific clinical symptoms, such as:
⇰ Reduction of daily feed consumption and body weight gain.
⇰ Worse feed conversion rate and carcass quality associated with hepatic lesions and overall sickly appearance.
In swine, Fumonisins are mostly associated with porcine pulmonary oedema (PPE) and symptoms are shown in Figure 4.
Numerous studies have shown that acute Fumonisin toxicity in swine is linked to pulmonary edema, which generally occurs between 3 and 5 days after the initial consumption of contaminated feed.
Figure 4. Different phases of Porcine pulmonary oedema syndrome in pigs induced by Fumonisin intoxication.
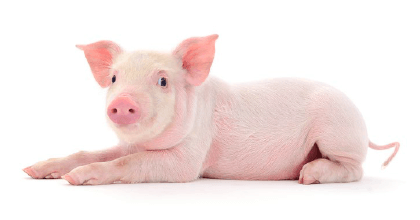
The effects of Fumonisins in poultry
Despite the relative resistance to Fumonisins of poultry, these mycotoxins can still have negative effects (Figure 5).
The most important effects of Fumonisins in poultry are:
- ⇰ Reduced thymus weight
- ⇰ Reduced immunization against Newcastle disease
- ⇰ Reduced macrophage numbers
- ⇰ Decreased lymphocyte response to Salmonella gallinarum infections
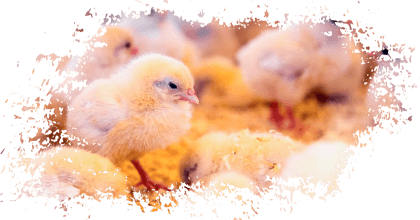
Figure 5. The effects of Fumonisins in poultry.
REMEDIATION OF FUMONISINS USING MINAZEL PLUS® – IN VITRO AND IN VIVO
A significant number of strategies have been adopted at pre- and post-harvest levels to reduce the mycotoxin menace in animal feeds worldwide.
A common method is the use of nutritionally inert organic (yeast cell walls and glucomannans) or inorganic (clay minerals such as aluminosilicates, bentonite, and zeolite) compounds as adsorbents that bind mycotoxins in the gastrointestinal tract of animals and reduce their bioavailability.
Recently, another category of mycotoxin biotransforming agents of biological origin (bacteria, fungi, enzymes, and plants) is becoming widely utilized (Loi et al., 2017).
Minazel Plus® in vitro adsorption and desorption studies
Minazel Plus® is a product based on organic modification of clinoptilolite.
⇰ It has been studied at pH 3.0 for adsorption (ADS) and at pH 6.5 for desorption (DES) using LC-MS/MS.
The results for ADS and DES of Minazel Plus® are shown in Table 3.
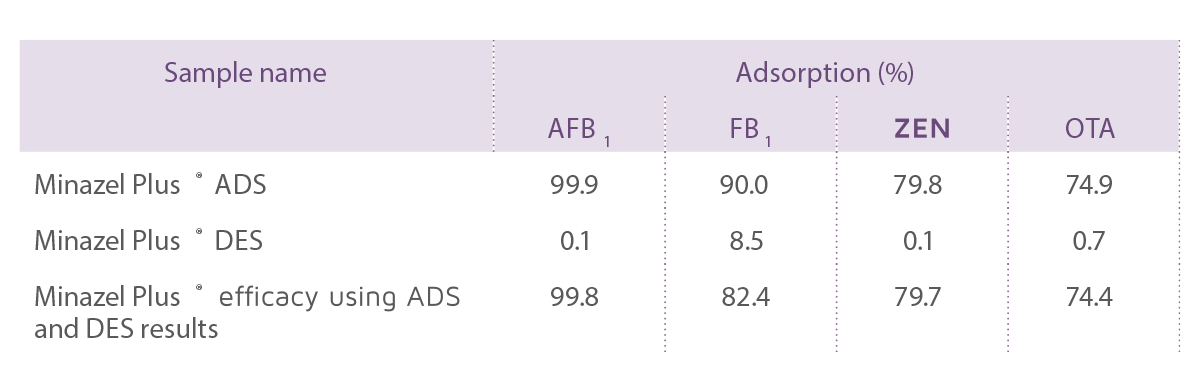
In vivo study with Minazel Plus® against Fumonisins in weaned piglets
To prove the efficacy of Minazel Plus®, an in vivo study was conducted on piglets.
The objective of this study was to evaluate the ability of a modified clinoptilolite zeolite-based substance (Minazel® Plus, Patent Co DOO, Misicevo, Serbia) to prevent gastrointestinal absorption of FB1 in piglets that received diets contaminated with Fumonisins.
This study was conducted by Dr. Panagiotis Tassis, DVM, Ph.D. Lecturer (Swine Medicine and Reproduction), School of Veterinary Medicine at Aristotle University of Thessaloniki, Greece in 2016.
⇰ The study was conducted on a total of 24 piglets (28 days of age) divided in 4 groups (6 animals/ group), each with equal number of male and female pigs. The total trial period was 42 days.
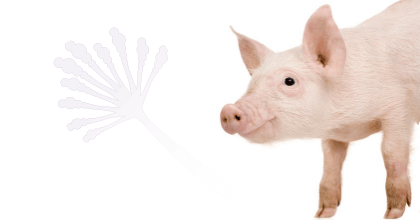
The trial groups and their relative interventions were as follows:
- ⇰ Control group: piglets fed a control diet without the addition of Minazel Plus® or FB.
- ⇰ Min 1 group: piglets that received control feed with the addition of 10 ppm of FB, without the inclusion of Minazel Plus®.
- ⇰ Min 2 group: piglets that received control feed with the addition of 10 ppm FB and 2 kg Minazel Plus®/ton of feed.
The piglets were allocated in separate autonomous parts of the experimental station that fulfilled all biosafety and welfare requirements.
Feed (well-balanced maize/wheat/barley mixture) was offered ad libitum to the trial animals.
Fumonisins were introduced into the feed by replacing the control maize with naturally contaminated maize.
At the end of the trial, the following results were registered regarding the inclusion of Minazel Plus® in the feed:
EFFECTS ON PRODUCTION PARAMETERS
⇰ Absence of significant differences of productive parameters mean levels in comparison with the control group.
⇰ Absence of significant differences in ADG between control group and 10 ppm FB1-fed piglets + Minazel Plus® for 42 days (Figura 6).
Figure 6. Mean average daily gain (kg) – total trial period.
⇰ Absence of significant differences in FCR between controls and 10 ppm FB1 fed piglets + Minazel Plus® for 42 days (Figura 7).
⇰ Absence of significant differences of liver enzymes mean levels in comparison with the control group.
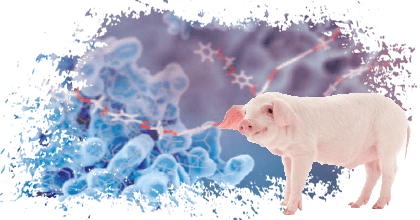
Figure 7. FCR (F:G) – Total trial period.
HISTOLOGICAL EXAMINATION
Histological features showed that normal limits were observed in liver, lungs, and intestine of all the examined animals (Image 2).
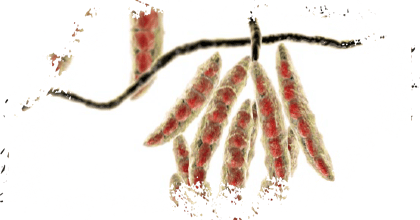
Image 2. Histological features (Hematoxylin and Eosin. Bar: 100 μm) of liver, lung, and ileum. Group A= Control; Group B = Min 1 group; Group C/D = Min 2 group.
These results allowed us to draw the following conclusions:
There was an absence of significant differences in ADG between the control group and the group that received 10 ppm FB1 + Minazel Plus® for 42 days.
There was an absence of significant differences in FCR between the control group and the group that received 10 ppm FB1 + Minazel Plus® for 42 days.
Histological features that were within normal limits were observed in liver, lungs, and intestines of all the examined animals.
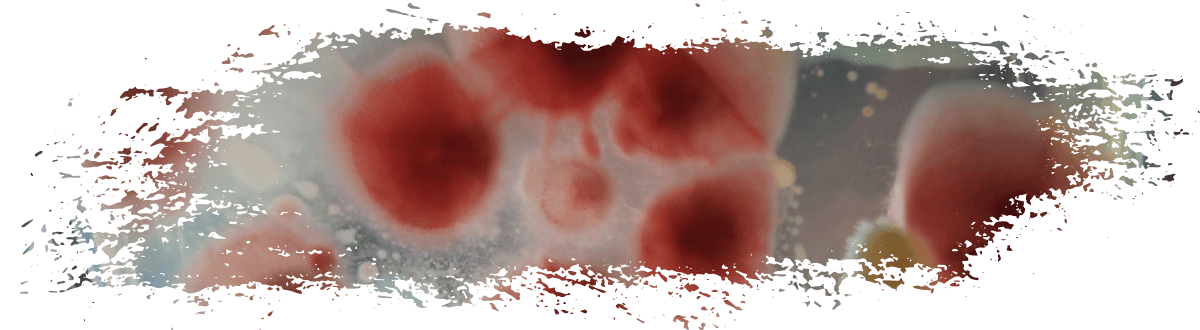
CONCLUSIONS
According to recent surveys, 96% of samples are contaminated with one or more than one mycotoxin and Fumonisins are the most frequently occurring mycotoxins globally.
The data from these surveys have shown the co-occurrence of two or more than two mycotoxins in corn and final feed materials.
The co-occurrence of more than one mycotoxin can lead to additive or synergistic effects when fed to sensitive animal species (e.g., swine and poultry amongst others).
⇰ This suggests that technologies are required for the control of mixtures of such contaminants in feedstuffs predominantly based on corn to minimize impacts on animal development.
Fumonisins are produced by several species of Fusarium, with Fusarium
moniliforme recognized as the main producer of this mycotoxin.
Fumonisins are highly soluble and have a greater capacity to spread easily, contaminating high volumes of water and feed.
Among animals, pigs are the most sensitive to Fumonisins, but they also affect poultry and cows.
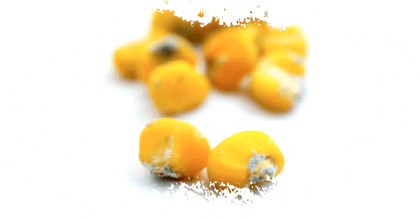
REFERENCES
Albertino, L.G. et al. (2021) ‘Clinical findings of equine leukoencephalomalacia’, Pesquisa Veterinária Brasileira, 41. doi:10.1590/1678-5150-pvb-6912.
Binder, E.M. Managing the risk of mycotoxins in modern feed production. Animal Feed Science and Technology 2007, 133 (1–2), 149-166.
Bin-Umer, M.A.; McLaughlin, J.E.; Basu, D.; McCormick, S.; Tumer, N.E. Trichothecene mycotoxins inhibit mitochondrial translation-implication for the mechanism of toxicity. Toxins 2011, 3(12), 1484-501. doi: 10.3390/toxins3121484.
Cavret, S.; Lecoeura, S. Fusariotoxin transfer in animal. Food and Chemical Toxicology 2006, 44(3), 444-453
Coffey, R. EndaCummins; ShaneWard. xposure assessment of mycotoxins in dairy milk. Food Control 2009, 20 (3), 239-249.
Colvin, B.M.; Cooley, A.J.; Beaver, RW. Fumonisin toxicosis in swine: clinical and pathological findings. J. Vet. Diagn. Invest. 1993, 5, 232–241.
Creppy, E.E. Update of survey, regulation and toxic effects of mycotoxins in Europe. Toxicol Lett. 2002, 28, 127(1-3), 19-28. doi: 10.1016/s0378-4274(01)00479-9.
EFSA Panel on Contaminants in the Food Chain (CONTAM); Scientific Opinion on Ergot alkaloids in food and feed. EFSA Journal 2012, 10(7), 2798. [158 pp.] doi:10.2903/j.efsa.2012.2798.
Giannitti, F.; Diab, S.; Pacin, A.; Barrandeguy, M.; Larrere, C et al. Equine leukoencephalomalacia due to fumonisins B1 and B2 in Argentina. Pesq Vet Bras. 2011, 31, 407-412.
Hamid, A.S.; Tesfamariam, I.G.; Zhang, Y.; Zhang, Z.G. Aflatoxin B1-induced hepatocellular carcinoma in developing countries: geographical distribution, mechanism of action and prevention. Oncology letters 2013, 5, 1087-1092
Haschek, W.M.; Gumprecht, L.A.; Smith, G.; Tumbleson, M.E.; Constable, P.D. Fumonisin toxicosis in swine: an overview of porcine pulmonary edema and current perspectives. Environ Health Perspect. 2001,109 (Suppl 2), 251-7. doi: 10.1289/ehp.01109s2251. PMID: 11359693; PMCID: PMC1240673.
Haschek, W.M.; Voss, K.A. Mycotoxins. Haschek and Rousseaux’s Handbook of Toxicologic Pathology. Third Edition. University of Illinois, Urbana, IL, USA, 2 USDA Agricultural Research Service, Athens, GA, USA, 2013; 1187-1258. http://dx.doi.org/10.1016/B978-0-12-415759-0.00039-X
IARC (International Agency for Research on Cancer). IARC Monographs on the Evaluation of Carcinogenic Risks to Humans, vol. 82. Lyon, International Agency for Research on Cancer, 2002; 82.
Khazaeli, P.; Najafi, M.L.; Bahaabadi, GA.; Shakeri, F.; Naghibzadeh tahami, A. Evaluation of aflatoxin contamination in raw and roasted nuts in consumed Kerman and effect of roasting, packaging and storage conditions. Life Sci. J. 2014, 10, 578–583.
Kovacić, S.; Pepeljnjak, S.; Petrinec, Z.; Klarić, M.S. Fumonisin B1 neurotoxicity in young carp (Cyprinus carpio L.). Arh Hig Rada Toksikol. 2009, 60(4), 419-26. doi: 10.2478/10004-1254-60-2009-1974. PMID: 20061242.
Kuroda, K.; Hibi, D.; Ishii, Y.; Yokoo, Y.; Takasu, S.; Kijima, A.; Matsushita K.; Masumura K.I.; Kodama Y.; Yanai T.; Sakai H.; Nohmi T.; Ogawa, K.; Umemura, T. Role of p53 in the progression from ochratoxin A-induced DNA damage to gene mutations in the kidneys of mice. Toxicological Sciences 2015, 144, 65-76
Liu, X.; Fan, L.; Yin, S.; Chen, H.; Hu, H. Molecular mechanisms of fumonisin B1-induced toxicities and its applications in the mechanism-based interventions. Toxicon. 2019, 167, 1-5. doi: 10.1016/j.Toxicon.2019.06.009.
Mathur, S.; Constable, P.D.; Eppley, R.M.; Waggoner, A.L.; Tumbleson, M.E.; Haschek, W.M. Fumonisin B(1) is hepatotoxic and nephrotoxic in milk-fed calves. Toxicol Sci. 2001, 60(2), 385-96. doi: 10.1093/toxsci/60.2.385. PMID: 11248152.
McLean, M.; Dutton, M.F. Cellular interactions and metabolism of aflatoxin: an update. Pharmacology and Therapeutics 1995, 65, 163-192.
Milićević, D.R.; Skrinjar, M.; Baltić, T. Real and perceived risks for mycotoxin contamination in foods and feeds: challenges for food safety control. Toxins 2010, 4, 572-92. doi: 10.3390/toxins2040572.
Pandya, J.P.; Arade, P.C. Mycotoxin: a devil of human, animal and crop health. Adv. Life Sci. 2016, 5, 3937–3941.
Pestka, J.J.; Zhou, H.R.; Moon, Y.; Chung, Y.J. Cellular and molecular mechanisms for immune modulation by deoxynivalenol and other trichothecenes: unraveling a paradox. Toxicology Letters 2004,153, 61-73.
Pleadin, J.; Frece, J.; Markov, K. Mycotoxins in food and feed. Adv Food Nutr Res. 2019, 89, 297-345. doi: 10.1016/bs.afnr.2019.02.007.
Pleadin, J.; Kovačević, D.; Peršia, N. Ochratoxin A contamination of the autochthonous dry-cured meat product “Slavonski Kulen” during a six month production process. Food Control 2015, 57, 377-384
Pleadin, J.; Staver, M.M.; Vahčić, N.; Kovačević, D.; Milone, S.; LaraSaftićb Scortichini, G. Survey of aflatoxin B1 and ochratoxin A occurrence in traditional meat products coming from Croatian households and markets. Food Control 2015, 52, 71-77
Pleadin, J.; Vahcic, N.; Persi.; Sevelj.; Markov, K.; Frece, J. Fusarium mycotoxins’ occurrence in cereals harvested from Croatian fields. Food Control 2013, 32, 49-54
Raj j, Farkaš, H, Jakovčević, Zdenka, Medina, A, Magan, A, Čepela R and Vasiljević M (2022) Comparison of multiple mycotoxins in harvested maize samples in three years (2018-2020) in four continents. Food Additives and Contaminants Part A, Mar;39(3):599-608.
Ringot, D.; Chango, A.; Schneiderb, Y.J.; Larondelle, Y. Toxicokinetics and toxicodynamics of ochratoxin A, an update. Chemico-Biological Interactions 2006, 159, 18-46
Sandmeyer, L.S.; Vujanovic, V.; Petrie, L.; Campbell, J.R.; Bauer, B.S.; Allen, AL.; Grahn, B.H. Optic neuropathy in a herd of beef cattle in Alberta associated with consumption of moldy corn. Can Vet J. 2015, 56(3), 249-56. PMID: 25750444; PMCID: PMC4327135.
Sorrenti, V.; Di Giacomo, C.; Acquaviva, R.; Barbagallo, I.; Bognanno, M.; Galvano, F. Toxicity of ochratoxin A and its modulation by antioxidants: a review. Toxins 2013, 5, 1742-1766.
Speijers, G.J.; Speijers, M.H. Combined toxic effects of mycotoxins. Toxicol Lett. 2004, 153(1), 91-8. doi: 10.1016/j.toxlet.2004.04.046.
Thiel, P.G. A molecular mechanism for the toxic action of moniliformin, a mycotoxin produced by Fusarium moniliforme. Biochem Pharmacol. 1978, 27, 483-486.
Voss, K.A.; Riley, T.R.; Waes Gelineau-van, J. Fumonisin B1 induced neural tube defects were not increased in LM/Bc mice fed folate-deficient diet. Molecular Nutrition & Food Research, 2014, 58(6).
Wang, Y.; Zheng, W.; Bian, X.; Yuan, Y.; Gu, J.; Liu, X.; Liu, Z.; Bian, J. Zearalenone induces apoptosis and cytoprotective autophagy in primary leydig cells. Toxicology Letters 2014, 226 (2), 182–91.
Weidner, M.; Welsch, T.; Hübner, F.; Schwerdt, G.; Gekle, M.; Humpf, H.U. Identification and apoptotic potential of T-2 toxin metabolites in human cells. Journal of Agricultural and Food Chemistry 2012, 60, 5676-5684.
WHO (World Health Organization). Safety Evaluation of Certain Mycotoxins in Food. WHO Food Additive Series 47, Geneva, 2001.
Yang, L.; Tu, D.; Zhao, Z.; Cui, J. Cytotoxicity and apoptosis induced by mixed mycotoxins (T-2 and HT-2 toxin) on primary hepatocytes of broilers in vitro. Toxicon 2017, 129, 1–10. 10.1016/j.toxicon.2017.01.001
Zhang, A.; Cao, J.L.; Yang, B.; Chen, J.H. Zhang, Z.-T.; Li, S.-Y.; Fu, Q.; Hugnes, C.; Caterson, B. Effects of moniliformin and selenium on human articular cartilage metabolism and their potential relationships to the pathogenesis of Kashin-Beck disease. J. Zhejiang Univ. Sci. B. 2010, 11 (3), 200–208.