Professor Carlos Augusto Fernandes de Oliveira
Department of Food Engineering, Faculty of Zootechnics and Food Engineering, University of São Paulo, Pirassununga, São Paulo, Brazil.

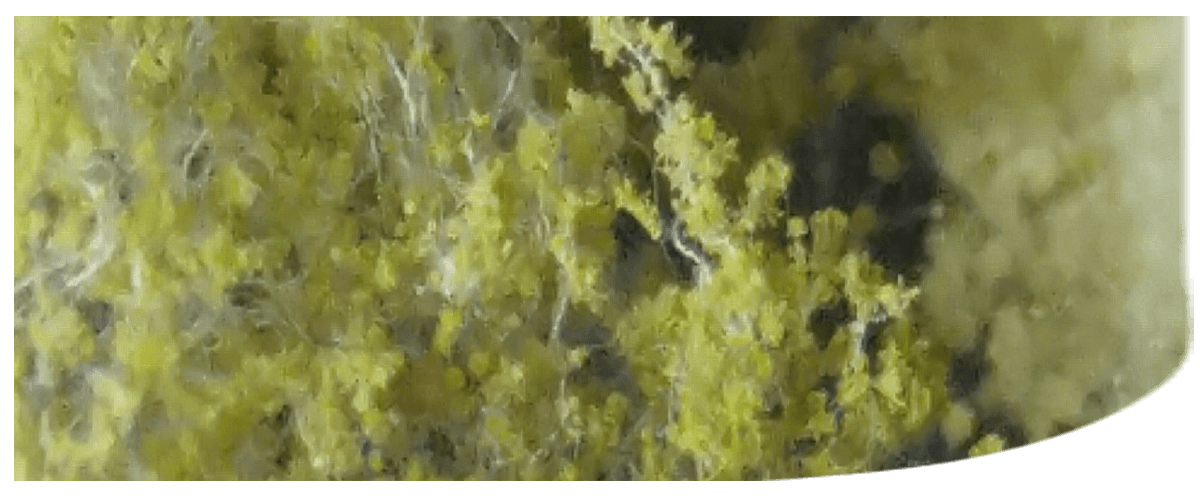
Micotoxins & Mycotoxicosis
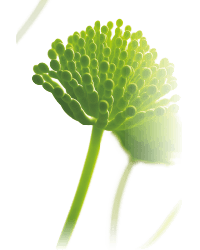
Mycotoxicosis is a disease associated with exposure to dietary mycotoxins, causing immune suppression and target organ toxicity with lesions mainly in liver, kidneys, epithelial tissue (skin and mucous membranes), and central nervous system, depending on the type of toxin.
The main groups of toxigenic fungi and their respective mycotoxins belong to the genus:
- Aspergillus:
- ⇰ A. flavus, A. parasiticus y A. nomius: aflatoxins
- ⇰ Fusarium: fumonisins, trichothecenes, moniliformins and zearalenone
- ⇰ Aspergillus ochraceus
- Penicillium: ochratoxins
Importantly, while food & feedstuffs may contain individual mycotoxins, contamination by multiple mycotoxins in these products is quite common and has become an important human and animal health concern due to the possible combined effects of different mycotoxins.
Toxicity of some individual mycotoxins may be increased in a synergistic, additive, or antagonistic way, when they occur as cocontaminants and are ingested by different animal species.
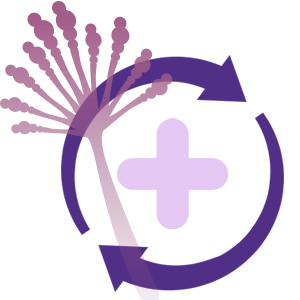
AFLATOXINS
Among the mycotoxins affecting farm animals, the aflatoxins are hepatotoxic, teratogenic and genotoxic compounds, also classified as carcinogenic to humans (Group 1) by the International Agency for Research on Cancer.
The aflatoxins were identified in 1961, aflatoxin B1 (AFB1) being the main type of toxin produced by Aspergillus under natural conditions.
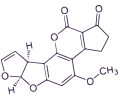
OCHRATOXIN A
Ochratoxin A (OTA) is an important nephrotoxic mycotoxin with immunotoxic, teratogenic, carcinogenic and perhaps neurotoxic effects causing liver and kidney cancer in numerous animal species.
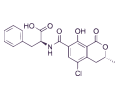
FUMONISINS
28 structurally related fumonisins have been isolated and identified, although fumonisin B1 (FB1) is the most predominant and toxic form produced by the fungi.
FB1 has been shown to be hepatotoxic, nephrotoxic and carcinogenic in several animal studies, also causing species-specific diseases including porcine pulmonary edema and equine leukoencephalomalacia.
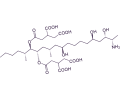
ZEARALENONE
Zearalenone (ZEN) is a mycotoxin which binds competitively to estrogen receptors, leading to estrogenic abnormalities and generative syndromes, especially in pigs.
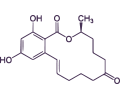
DEOXYNIVALENOL
Deoxynivalenol (DON), correspondingly named vomitoxin because of its emetic effects after ingestion, is another mycotoxin produced by Fusarium species, which belongs to the class B trichothecenes and often co-exist with ZEN in feed materials such as corn, oats, barley, and wheat.
The acute exposure to DON also causes abdominal pain, salivation, diarrhea, leukocytosis, and gastrointestinal hemorrhage (Oliveira et al., 2014).
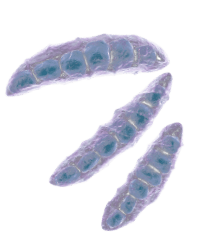
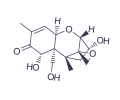