Al-Zahraa Mamdouh1† and Eman Zahran2*†
1Fish Diseases Department, National Institute of Oceanography and Fisheries
(NIOF), Egypt
2Department of Aquatic Animal Medicine, Faculty of Veterinary Medicine, Mansoura University, Mansoura 35516, Egypt
†These authors share first authorship
*Corresponding author: [email protected]
ORCID: 0000-0003-2212-3688
Limited availability and rising costs of dietary fishmeal are among the biggest challenges faced by the shrimp aquaculture industry.
⇰ To overcome this, the use of plant-based ingredients instead of fishmeal in finished shrimp feeds has been on the rise and with success.
However, using crops in feed increases the risk of contamination with fungi and mycotoxins, as well as the incidence of mycotoxicosis in shrimp.
Mycotoxicosis negatively influences body weight, growth, disease resistance, and survivability in shrimp, leading to reduced aquaculture productivity.
Furthermore, bioaccumulation of mycotoxins can pose a risk to humans through consumption of shrimp, which means that it is a threat to food security and public health.
Additionally, mycotoxins are genotoxic, carcinogens, and immunosuppressors.
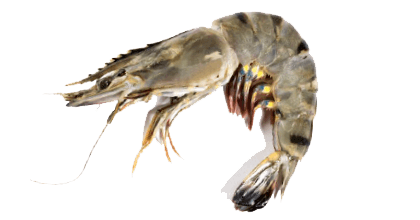
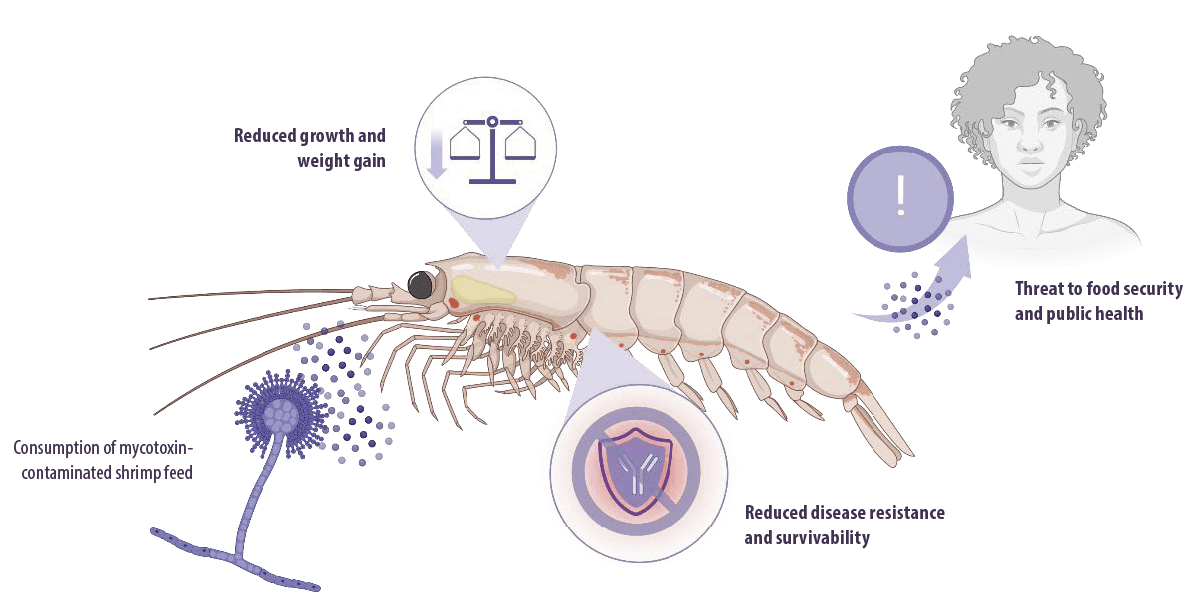
Even though research on mycotoxins has been going on since the 1960s, information about mycotoxicosis in shrimp is still lacking.
Thus, efforts should be made to monitor their level of contamination with mycotoxigenic fungi and mycotoxins.
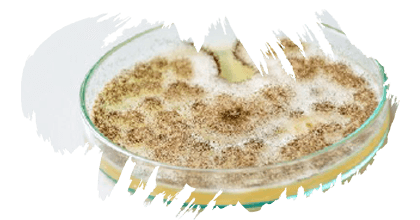
Mycotoxins in shrimp feed
Source of contamination
Mycotoxins are secondary metabolites produced by filamentous fungi that contaminate a wide variety of food and feed products worldwide (Marroquín-Cardona et al., 2014).
Hundreds of mycotoxins are known. Among them, aflatoxins (AF), citrinin, patulin, penicillic acid, ochratoxin A (OTA), deoxynivalenol (DON), fumonisins (FUMS), and zearalenone (ZEN) are the most common contaminants in cereal grains, and most of them are produced by the three fungal genera, Aspergillus, Penicillium and Fusarium (Ismaiel y Papenbrock, 2015).
⇰ These fungi can infect a variety of crops and agricultural products, including wheat, rice, maize, nuts, corn, soybean, and sorghum, affecting many agricultural commodities before and after harvest, as well as finished feeds (Pleadin et al., 2019).

According to the Food and Agriculture Organization (FAO), in 2010, global farmed shrimp production was estimated to be nearly 3.78 million tons, with production increasing over three-fold from 1.1 million tons in 2000, with an average annual growth rate of 14.5% since 1950.
The most commonly cultured shrimp species are White-leg shrimp (Litopenaeus vannamei) and Black tiger prawn (Penaeus monodon). Other minor cultivated species include Kuruma prawn (Penaeus japonicus) and Fleshy prawn (Penaeus chinensis), Indian white prawn (Penaeus indicus) and Banana prawn (Fenneropenaeus merguiensis) (Tacon et al., 2013).
White-leg shrimp (L. vannamei) was ranked first in 2010 in total farmed fish and crustacean production, with a total value of US$11.23 billion.
Shrimps have a fast growth rate, short culture period, high export value, and high market demand. As a result, the industry has rapidly grown worldwide.
However, shrimp farming faces many challenges, including the high costs of feeding programs (Ayisi et al., 2017).
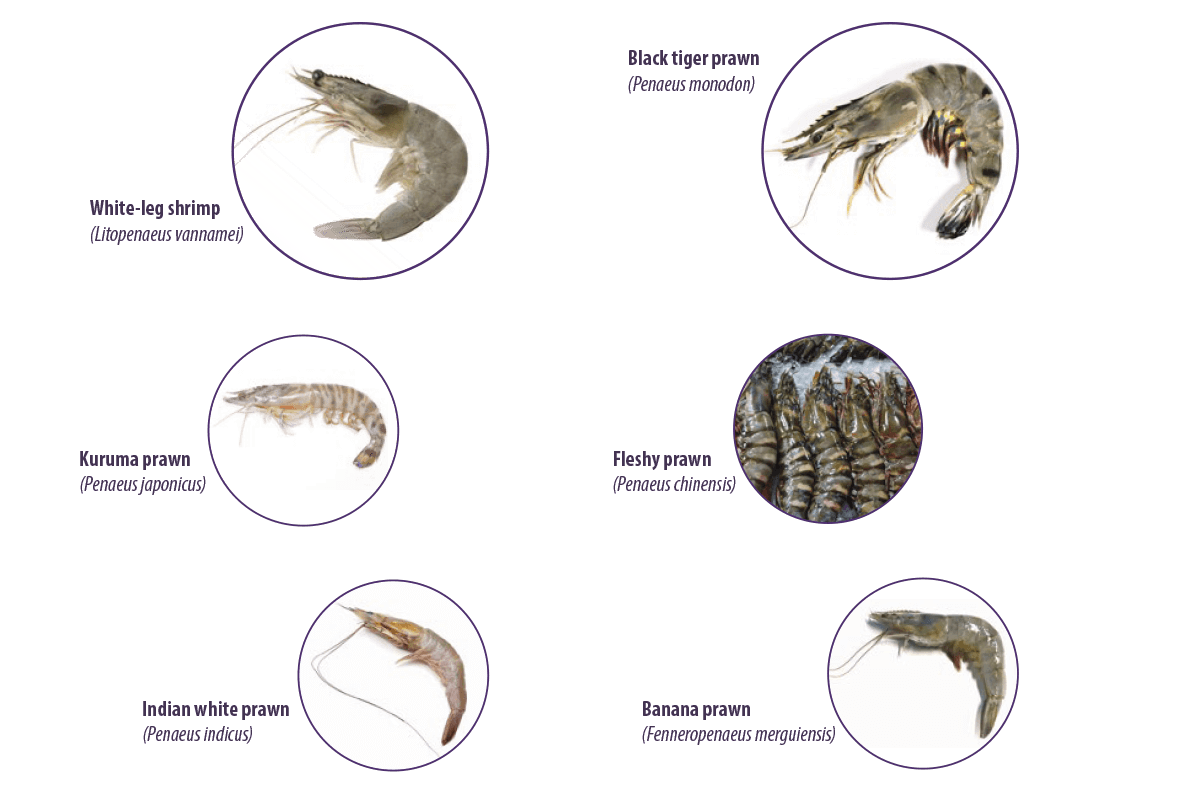
Commercial compound feed is mainly required for shrimp aquaculture to provide the necessary nutrients for growth and health maintenance (Oliveira y Vasconcelos, 2020).
40.0% of all farmed fish, including shrimp, require a large amount of protein-rich aquafeeds (Tacon y Metian, 2008).
Proteins are the most expensive nutrients in diets for shrimp culture (Ayisi et al., 2017).
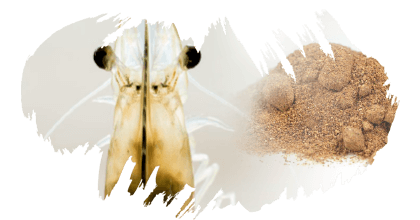
Fish meal is the most used protein source in commercial shrimp feed due to its high digestibility, essential amino acids, and fatty acid profile (Oujifard et al., 2012).
⇰ In 2008, shrimp industry consumed 27.2% of the fish meal used in aquafeeds, making it the largest consumer of this source of protein (Ayisi et al., 2017).
However, the increased demand for aquaculture production has resulted in an increased demand for fish meal and fish oil and the subsequent shortage of pelagic fish and other fish species used in their production (Oliveira y Vasconcelos, 2020).
⇰ Therefore, the limited availability and rising costs of dietary fish meal have resulted in the increased use of plant proteins as alternatives to fish meal and fish oil in shrimp and fish feeds (Katya et al., 2016).
Over the past decades, different plant protein sources have been studied to replace fish meal in shrimp feed, including soybean meal (Bulbul et al., 2015; Sharawy et al., 2016; Yang et al., 2015), canola meal, peanut meal (Bulbul et al., 2014), rice meal (Macias-Sancho et al., 2014), and sunflower oil cake (Dayal et al., 2011).
However, plant-based shrimp feed is susceptible to contamination with various fungi and mycotoxins.
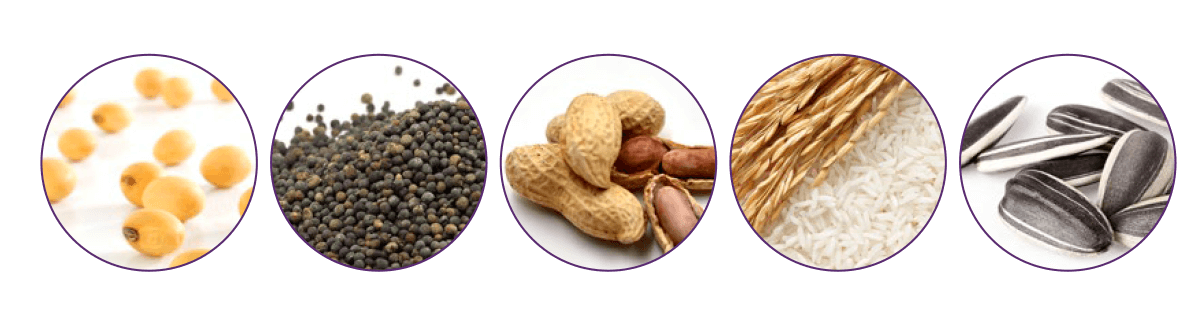
The presence of mycotoxins in commercial shrimp feed has been reported in different locations around the world.
The oldest documented survey of mycotoxin occurrence was in black tiger shrimp (P. monodon) finished feed in the Philippines (Bautista et al., 1994).
Aflatoxins were detected in commercial shrimp feed in the Eastern and Southern regions of Thailand (Bintvihok et al., 2003).
In Thailand, shrimp and fish feed samples were contaminated with zearalenone and OTA, whereas in India, shrimp feed was contaminated with AF (Fegan y Spring, 2007).
In 2014, fish and shrimp feed samples from Europe (Croatia and Portugal) and South East Asia (Singapore, India, Thailand, and Myanmar) were analyzed for the detection of AF, ZEN, DON, FUM, and OTA (Gonçalves et al., 2018a), and the results showed that a higher occurrence of FUM was found in European samples than in SE Asia.
- ⇰ The remaining mycotoxins showed similar occurrence average and maximum levels for Europe and SE Asia, with mycotoxins being detected in all analyzed samples.
- ⇰ In addition, in Europe, 50% of the samples had more than one mycotoxin per sample, and in Asia, 84% of the samples were contaminated with more than one mycotoxin.
In 2015, samples of fish and shrimp feed in Europe (Denmark, Austria, Netherlands, and Germany) and SE Asia (Vietnam, Indonesia, and Myanmar) showed high DON contamination and a higher co-occurrence risk in both regions (Gonçalves et al., 2017).
In 2016, shrimp feed from India and fish feed from Indonesia, Myanmar, Taiwan, and Thailand showed mycotoxin contamination, with fish feed samples showing lower contamination than shrimp feed and relatively high contamination of DON in shrimp feed (Gonçalves et al., 2018b).
The effects of mycotoxins on shrimp health
Aflatoxins
Mechanisms and effects on shrimp health
Aflatoxins are the most commonly found mycotoxins in shrimp feed, mainly produced by Aspergillus flavus and Aspergillus parasiticus, and to a minor extent, by Aspergillus nomius and Aspergillus bombycis, Aspergillus pseudotamari and Aspergillus ochraceoroseus (Varga et al., 2011).
They are classified according to their blue or green fluorescence under ultraviolet light in AFB1, AFB2, AFG1 and AFG2 (Dhanasekaran et al., 2011), appearing in a variety of feedstuffs, including maize, ground nuts, rice and sorghum cottonseed, spices, cereals, soybean, cocoa, and meat (Patriarca and Pinto, 2017; Vila-Donat et al., 2018).
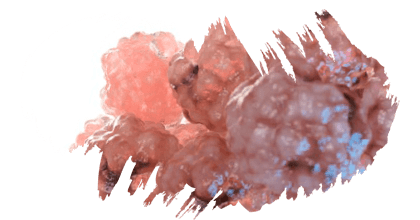
Aflatoxins induce toxicity during their metabolism in the liver through the generation of intracellular reactive oxygen species (ROS), such as superoxide anions, hydroxyl radicals, and hydrogen peroxide (H2O2), under the action of cytochrome P450.
- ⇰ These ROS target cellular DNA, RNA, proteins, and cell membranes, leading to impaired cell function, oxidative stress, DNA damage, cytolysis, and apoptosis (Asim et al., 2011; Yang et al., 2016).
- ⇰ They can also target the p53 tumor suppressor gene, which causes hepatocarcinoma (Kew, 2013).
Aflatoxin metabolism mainly occurs in the liver under the action of cytochrome P450 in electrophilic reactive epoxides.
- ⇰ Epoxide metabolites bind to DNA, RNA, and cellular macromolecules in the liver (Abrar et al., 2013).

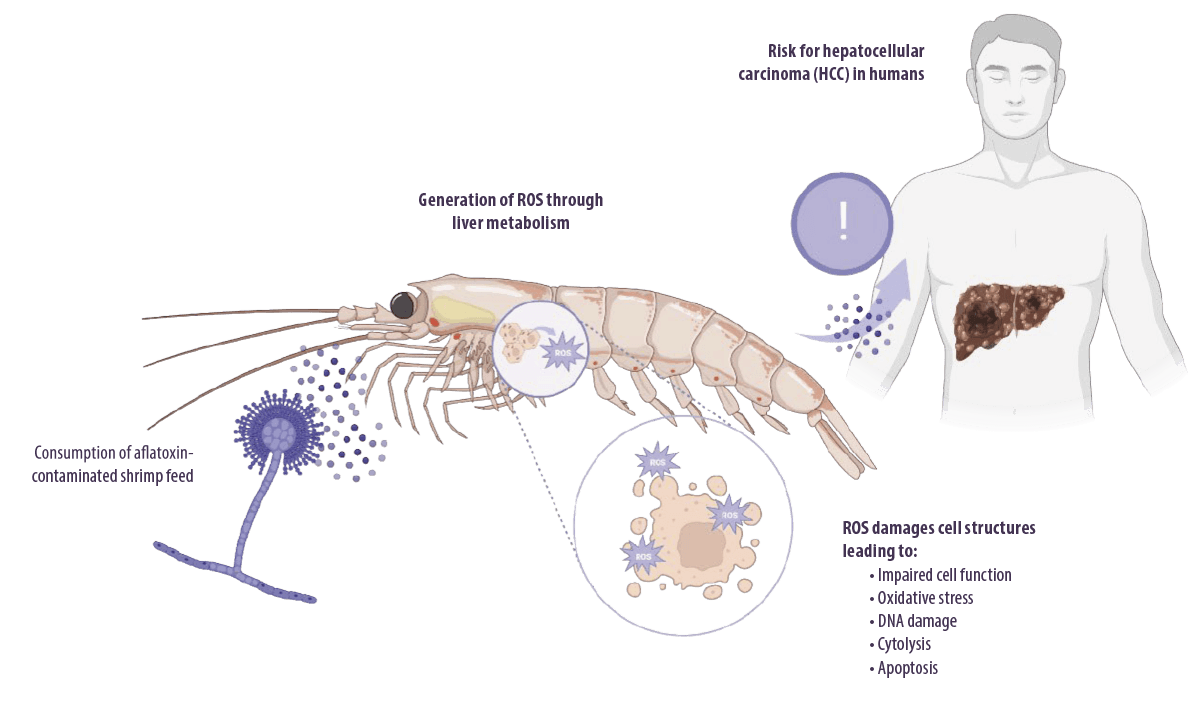
AFLATOXICOSIS IN HUMANS
AFB1 is the most potent experimental hepatocarcinogen known in humans (Humans and Cancer, 2002), although no animal model exposed to the toxin developed HCC.
⇰ TaIt also accounts for approximately 9.2% of all new cancers worldwide (Ferlay et al., 2010). Other liver diseases, including cirrhosis (Humans, 2014) and hepatomegaly (Gong et al., 2012), have also been associated with aflatoxin toxicity in humans.
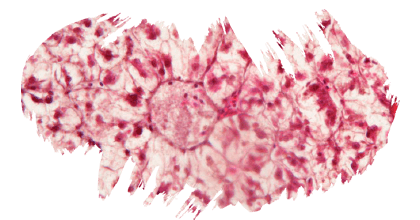
AFLATOXICOSIS IN FISH & SHRIMP
Impaired liver function and oxidative stress are the expected consequences of aflatoxicosis in fish and experimental animals.
Aflatoxins can cause severe health problems and reduce the yield of shrimp cultures.
In shrimp, AFB1 has been extensively reported to affect:
- ⇰ Growth performance
- ⇰ Blood parameters
- ⇰ Histological parameters
- ⇰ Antioxidant enzyme activity
- ⇰ The expression of transcriptome- and immune-related genes in the hepatopancreas
The hepatopancreas is the primary organ affected by dietary AFB1 as it is the main digestive organ and immune system in shrimp (Pérez-Acosta et al., 2016) and its functions are related to the synthesis and secretion of digestive enzymes and immune molecules, absorption of nutrients, storage of reserves, and excretion (Zhao et al., 2017).
Moreover, many studies have also reported that AFB1 can damage the intestinal mucosal barrier and induce an imbalance in the intestinal microbial populations (Fang et al., 2020; Wang et al., 2018).
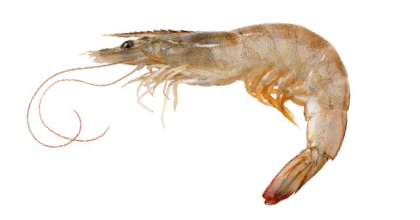
Several studies have reported the effects of AFB1 on shrimp growth and immune response. For example:
Exposure of L. vannamei to AFB1 (500 μg/kg AFB1) for 8 weeks significantly decreased final body weight (FBW), weight gain (WG, %), and significantly increased inducible nitric oxide synthase (iNOS) activity and glutathione (GSH) and malondialdehyde (MDA) levels in the hepatopancreas (Yu et al., 2018).
Similarly, juvenile L. vannamei fed a diet containing AFB1 for 42 days had significantly decreased mean weight, feed intake, growth rate, and nitrogen retention efficiency, and significantly increased enzymatic activity of alkaline phosphatase (ALP) and glutathione S transferase (GST) in the hepatopancreas (Tapia-Salazar et al., 2022).
In the same context, exposure of L. vannamei to AFB1 for 8 weeks significantly decreased weight gain (12000 µg AFB1/kg), total antioxidant capacity (2000 µg AFB1/kg), GST, superoxide dismutase (SOD), Na and K ATPase in hepatopancreas, and significantly increased GST in the hemolymph (1600 µg AFB1/kg) (Wang et al., 2012).
L. vannamei juveniles fed diets containing grains naturally contaminated with total aflatoxins (500, 1000, and 2000 µg/kg for 28 days) showed significantly decreased weight gain and feed intake, and significantly increased feed conversion ratio.
In addition, histological analysis of the hepatopancreas revealed that B-cell activity and mitotic cell activity were significantly decreased, whereas lipid storage, tubular epithelial atrophy, and hepatopancreatocyte sloughing were significantly increased (Tapia-Salazar et al., 2012).
F. indicus fed diets containing 1600 ppb AFB1 for 8 weeks showed significantly decreased final weight, survival rate, total hemocyte count, total plasma protein and phagocytic activity and significantly increased specific growth rate.
Histopathological examination of the hepatopancreas showed atrophied R-cells, severe necrosis and shrinkage of the tubules, infiltration of haemocytes and fibroblastic cells that produced a fibrosis-like exterior aspect, cell degeneration and pyknotic nuclei in the lumen, spread necrosis and complete destruction of B cells, complete isolation of connective tissue from hepatopancreas tissue and in midgut tissue and separation between mucosal and submucosal layers (Ghaednia et al., 2013).
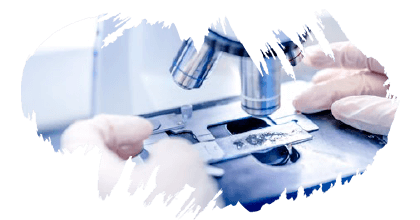
Ultrastructural examination of the hepatopancreas of P. monodon fed a diet containing 1000 and 2000 ppb AFB1 for 8 weeks showed nuclear vacuolation and condensation, appearance of electron-dense material, irregular shape of nucleus, loss of nuclear membrane, and fragmentation of the endoplasmic reticulum, as well as swelling of mitochondria, vacuolation and loss of organelles in a few areas, formation of vesicles in the cytoplasm, loss of microvilli, cell rounding, and extensive necrosis (Radhika et al., 2012).
Exposure of juvenile L. vannamei to 25, 50, 100, 500, and 1000 µg/kg of AFB1 induced a significant decrease in weight gain, specific growth rate, and a significant increase in survival rate, aspartate amino transferase (AST) and alanine amino transferase (ALT) levels, and serum total antioxidant activity (Zeng et al., 2016).
L. vannamei fed 15 ppm AFB1 for 8 days had a significantly decreased survival rate, significantly increased SOD, GST, glutathione peroxidase (GPx), and catalase (CAT) levels, and differential expression of 1024 genes involved in functions including peroxidase metabolism, signal transduction, transcriptional control, apoptosis, proteolysis, endocytosis, cell adhesion, and cell junction.
It also induced severe histological alterations in the shrimp hepatopancreas, including separation between the myoepithelial layer and the epithelium of the hepatopancreas, excess fat in many vacuolated cells, nuclear pyknosis, cell lysis, and cell necrosis (Zhao et al., 2017).
In a more recent study, exposure of L. vannamei to 168.3 μg/kg of AFB1 for 58 days significantly decreased weight gain, specific growth rate, survival rate, protein efficiency ratio, protein productive value, and AST and ALT levels in the serum and hepatopancreas, significantly increasing the feed conversion ratio. It also induced a significant increase in serum MDA level and GST and GPx activities, while significantly decreasing their activities in the hepatopancreas. In addition, it decreased the acid phosphatase (ACP) and alkaline phosphatase (AKP) levels in the serum and hepatopancreas.
Furthermore, AFB1 significantly downregulated the expression of immune-related genes, including myeloid differentiation factor 88 (MyD88), Dorsal, Tumor necrosis factor receptor-associated factor 6 (TRAF6), Relish, Domeless, cytochrome P450 and penaeidin 3a (Chen et al., 2020).
L. vannamei fed diets contaminated with 200 μg/kg of AFB1 for 42 days suffered from significantly reduced feed intake, growth rate, and nitrogen retention efficiency and significantly increased ALP and GST activity (García-Pérez et al., 2020).
Exposure of P. vannamei to 500 μg/kg of AFB1 for six weeks significantly decreased survival rates, weight gain, and the expression of immune-related genes in the intestine, including Rab, GST, mucin-like-PM, Dorsal, Relish and Pro-PO.
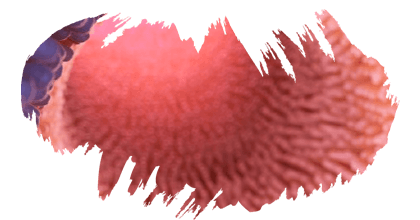
AFB1 induced damage to the antioxidant system and dysregulation of intestinal microbiota in L. vannamei fed a diet containing 5 ppm AFB1 for 30 days, as it induced a significant increase followed by a significant decrease in CAT, SOD, and GPX activities and a significant increase in MDA in the intestine and hepatopancreas.
In addition, an imbalance in intestinal microbiota was indicated at the phylum level, the relative abundance of Proteobacteria and Firmicutes increased, and the relative abundance of Bacteroidetes decreased.
At the genus level, the relative abundances of Vibrio and Photobacterium significantly increased, and the relative abundances of Flavobacterium_ sp_M and Tenacibaculum decreased (Wang et al., 2018).
Exposure of L. vannamei to 977.11 and 1605.61 μg/kg of AFB1 for 28 days induced significant increase in AST, ALT activities in hemolymph and the histological alternation index (HAI) value of hepatopancreas, with a significant decrease in total protein and fat (triglyceride and cholesterol) in hemolymph.
Histopathological examination of the hepatopancreas showed irregular and abnormal appearance of the hepatopancreas tubular structure, tubular cell vacuolation, separation of epithelium and myoepithelial layer, necrosis of hepatopancreatic cells and pyknotic nuclei, hepatopancreatic inflammation, and penetration of hemocytes into the space between tubules (Jamshidizadeh et al., 2019).
T-2 toxin
Mechanisms and effects on shrimp health
There is little information on the toxicity of other mycotoxins to aquatic invertebrates, but T-2 toxin has been reported to impair shrimp growth and immune responses in many studies.
Trichothecenes (mainly T-2 toxin) are well known for their capacity to inhibit eukaryotic protein synthesis by binding to the 60S subunit of eukaryotic ribosomes and inhibiting peptidyl transferase activity.
⇰ This leads to inhibition of the initiation, elongation, or termination of the chain elongation step in protein synthesis (Arunachalam y Doohan, 2013).
T-2 toxin can also:
- ⇰ Inhibit DNA and RNA synthesis (Minervini et al., 2004)
- ⇰ Alter cellular membrane structure (Diesing et al., 2011) and mitochondrial function
- ⇰ Arrest the cell cycle (Pestka, 2010)
- ⇰ Induce oxidative stress by increasing lipid peroxidation and altering antioxidant defenses, which eventually impairs protein synthesis and causes DNA damage (Doi and Uetsuka, 2011; Wu et al., 2014)
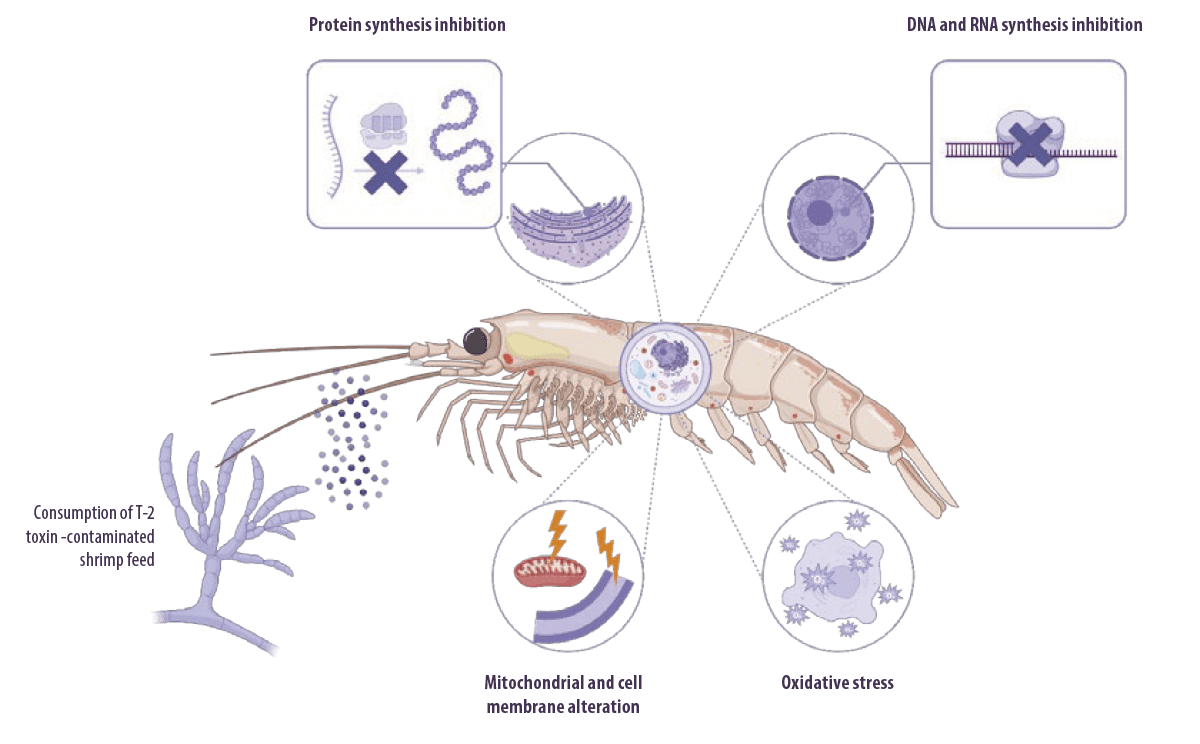
The effects of T-2 toxin on growth, antioxidant capacity and histopathological findings in the hepatopancreas of L. vannamei were investigated through dietary exposure to 0.5, 1.2, 2.4, 4.8, and 12.2 mg/kg of T-2 toxin for 20 days and it was reported that concentration of mT-2 in the hepatopancreas was significantly increased in a dose-dependent manner. In addition, growth and survival rates significantly decreased.
Moreover, MDA concentration was significantly increased at a dose ≥2.4 mg/kg of T-2 toxin, while SOD and GPx, total antioxidant capacity (T-AOC), and GSH content increased at a dose of 2.4–4.8 mg/kg of T-2 toxin but declined at dose 12.2 mg/kg.
Histopathological changes in the hepatopancreas were dose-dependent, with evident autophagy at the highest exposure dose (Deng et al., 2017).
T-2 toxin induced toxic effects on the hemolymph, immune system, and hepatopancreas of L. vannamei after dietary exposure to 0.5, 1.2, 2.4, and 4.8 mg/kg for 20 days, such as a significant reduction in weight gain, specific growth rate (at all doses) and survival rate (0.5 and 1.2 mg/kg). It significantly decreased phenoloxidase enzyme activity (all doses), hemocyte count (2.4 mg/kg) and albumin concentration (1.2, 2.4 and 4.8 mg/kg). Moreover, it altered the activities of the hemolymph enzymes GOT, GPT, and ALP in a dose-dependent manner.
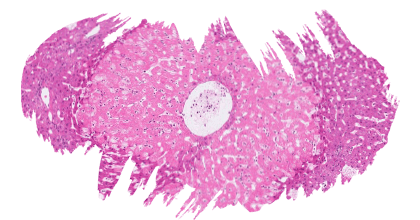
Exposure of L. vannamei to T-2 toxin at concentrations of 0.5, 1.2, 2.4, 4.8 and 12.2 mg/kg for 20 days significantly reduced the weight gain, specific growth rate, and survival rate.
Histopathological examination of the intestine showed concentration-dependent degenerative and necrotic changes, with initial inflammation of the mucosal tissue at 0.5 and 1.2 mg/kg, progressing to the disappearance of intestinal villi and degeneration and necrosis of the submucosa at 12.2 mg/kg.
Intestinal digestive enzymes protease and amylase significantly decreased with increasing concentrations of T-2 toxin, whereas lipase activity increased with higher T-2 toxin concentrations (Huang et al., 2019).
The effect of T-2 toxin on the muscle proteins of L. vannamei was investigated through dietary exposure to 1.2, 2.4, 4.8 and 12.2 mg/kg for 20 days and it was reported that the amount of myofibrillar protein, sarcoplasmic and stroma proteins increased at the low concentration of 1.2 mg/kg T2, while higher concentrations induced significant declines in a dose-dependent manner.

T-2 toxin affected the fatty acid, water distribution, and muscle histopathology of L. vannamei where exposure to 0.5, 1.5, 4.5 and 13.5 mg/kg for 20 days significantly affected the muscle fatty acid composition with an initial decrease in saturated fatty acid (ΣSFA), monounsaturated fatty acids (ΣMUFA), and polyunsaturated fatty acids (ΣPUFA), followed by an increase in the high-dose groups.
In addition, T-2 toxin significantly affected water distribution in the muscle, where high doses reduced free water content resulting in a reduction in the water holding capacity and, hence, changes in shrimp muscle quality (Bi et al., 2019).
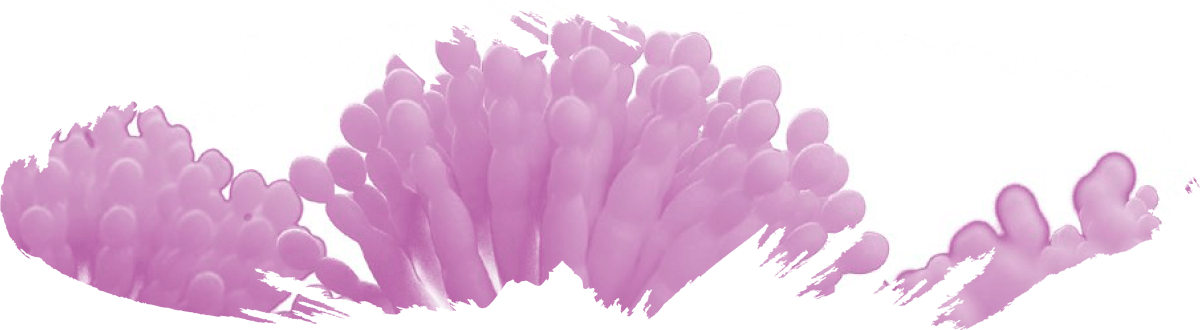
Deoxinivalenol, Fumonisins and Ochratoxin A
Effects on shrimp health
A few more studies have reported other mycotoxin effects on shrimp.
L. vannamei fed diets contaminated with 250, 500, and 1000 μg DON/kg for 5 weeks suffered from significantly decreased weight gain (1000 μg /kg) and significantly increased GST (500 μg /kg) and SOD activity (1000 μg /kg), while the gene expression of SOD and GPx were significantly decreased at doses of 500 and 1000 μg/kg DON. In addition, the hepatopancreas immune response related gene expression of HSP70, Toll 1 and Dorsal was higher at a dose of 250 μg DON/kg, and the expression of proPO, LGBP and PPAF was significantly higher at a dose of 1000 μg DON/kg.
Histopathological examination revealed that intestinal mucosal folds were impaired by apoptosis in intestinal epithelial cells, and B cell numbers and diameters of the hepatopancreas tubules were affected by different DON doses (Xie et al., 2018).
L. vannamei exposed to 0.5, 0.75 and 1.0 µg/g of FB1 for 18 days showed significantly decreased phenoloxidase, total hemocyte count, and superoxide anion rate.
The hepatopancreas showed histopathologic lesions, including deformation of the hepatopancreas tubules with a loss of normal cellular structure, presence of melanization, and the tubules showing severe vacuolization with cellular retraction (Mexía-Salazar et al., 2008).
Feeding P. monodon with a diet containing DON and OTA for 8 weeks significantly altered growth and immune parameters.
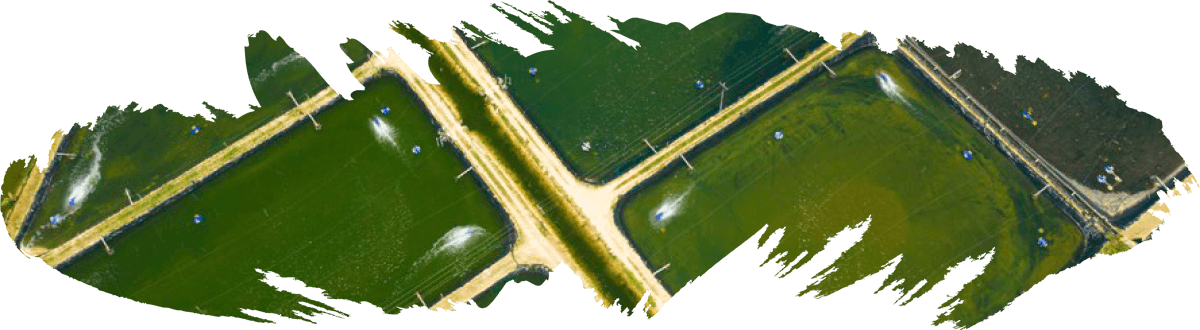
Protection of shrimp against mycotoxicosis
Various strategies have been developed to reduce the toxic effects of mycotoxins in feed, such as:
- ✓ Physical decontamination (Grenier et al., 2014; Pankaj et al., 2018)
- ✓ Chemical decontamination (Čolović et al., 2019)
- ✓ Biological decontamination (Shu et al., 2018)
Some of the compounds used in different studies to alleviate mycotoxin-induced toxicity in shrimp are as follows.
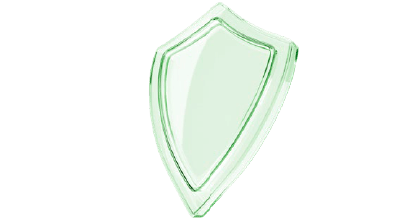
Probiotics
The use of probiotics has been shown to significantly improve the survival rate and growth performance of both aquaculture and terrestrial farmed animals (Wang et al., 2019; Zhang et al., 2019).
In shrimp aquaculture, probiotics improve the rate of digestibility and absorption and promote growth, healthy intestinal morphology and flora, a robust immune response, and resistance to diseases (Amoah et al., 2019; Azad et al., 2019; Duan et al., 2018; Zuo et al., 2019).
Supplementation of Lactobacillus pentosus HC-2 (5 × 108 CFU/g feed) to P. vannamei for 6 weeks alleviated AFB1-induced toxicity (500 μg/kg) by increasing the survival rate and percent weight gain, enhancing the intestinal morphology and community structure of intestinal microbiota by significantly increasing the abundance of Proteobacteria and decreasing the abundance of Firmicutes and Bacteroidetes. It also increased the expression of immune genes, including Rab, GST, mucin-like-PM, Dorsal and Pro-PO (Fang et al., 2020).
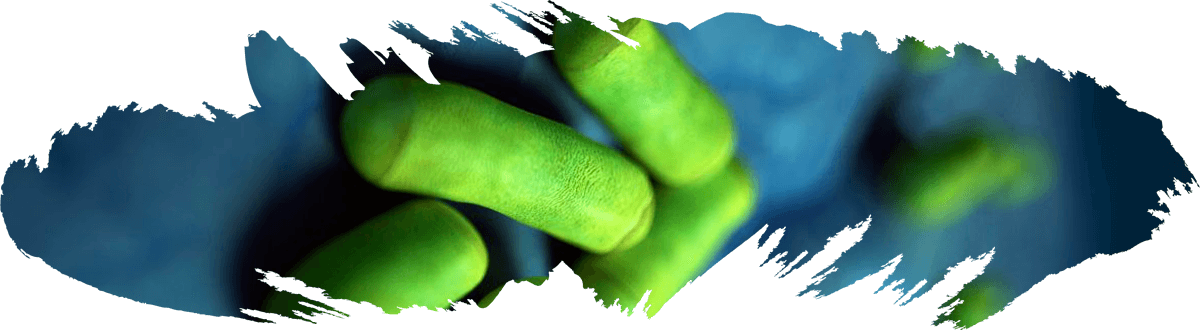
Antioxidants
Other studies involved the use of antioxidants.
Curcumin supplementation was beneficial to protect L. vannamei juveniles against immune toxicity caused by AFB1 (200 μg/kg).
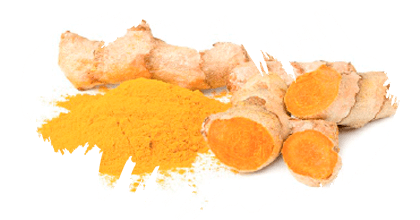
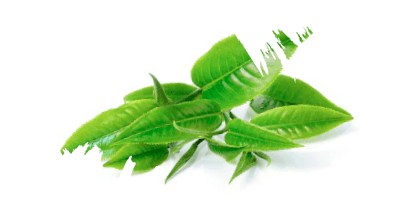
Tea polyphenols were effective in the protection of P. vannamei against the deterioration of shrimp muscle quality induced by AFB1 (1.2–2.7 mg/kg feed), where it inhibited the expansion of muscle fiber spaces and inflammation and induced a significant protective effect against the decrease in muscle nutrients and changes in the protein composition (Huang et al., 2021).
Quercetin, tea polyphenols, and rutin are widely used plant-derived antioxidants that possess antioxidant, antinflammatory, and antitumoral properties.
Adsorbents
The addition of clay to the diet of L. vannamei exposed to 200 μg/kg AFB1 for 42 days at doses of 4, 5, and 6 g/kg improved nitrogen retention and decreased ALP (6 g/kg) and GST (4 g /kg) activity (García-Pérez et al., 2020).
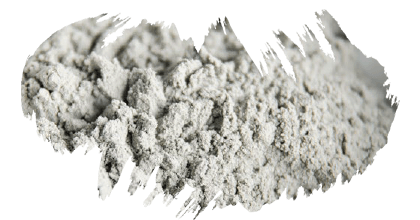
Detoxification
A new approach for the detoxification of mycotoxins in shrimp is the use of natural components, such as bile acids and myo-inositol.
Bile acids are detergent molecules synthesized from cholesterol in vertebrates (Šarenac and Mikov, 2018) and function as signaling molecules, regulating the detoxification and antioxidant system of mammals by activating nuclear hormone receptors (Baijal et al., 1998; Reschly et al., 2008).
Myo-inositol is a vitamin-like essential nutrient that has been shown to enhance the enzymatic antioxidant capacity in carp. However, in shrimp, myo-inositol slightly (but not significantly) mitigated the negative impacts to the growth, antioxidant enzyme activities, and immune-related gene expression of L. vannamei caused by AFB1 exposure (Chen et al., 2020).
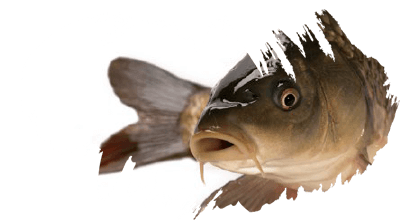
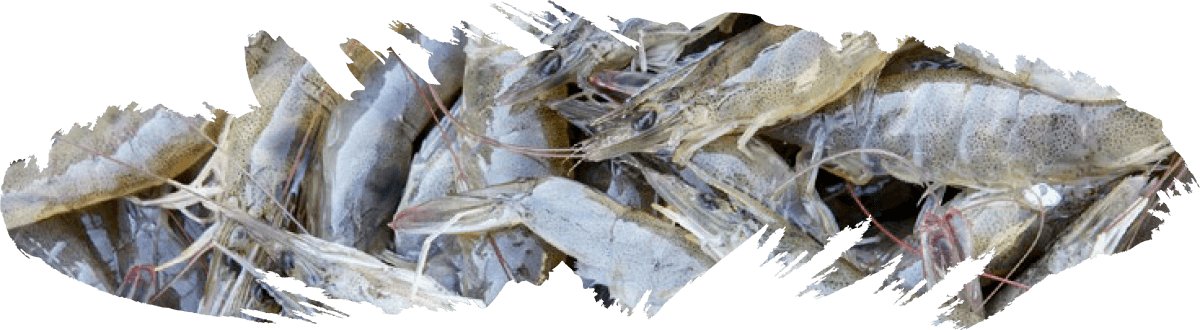
CONCLUSIONS
Increasing plant-based ingredients in aquafeed is considered one of the challenges in shrimp aquaculture due to the higher susceptibility to fungal contamination and mycotoxin production, which eventually impacts shrimp productivity.
Mycotoxins affect shrimp health through a variety of mechanisms that interfere with the normal physiological body functions.
⇰ Different approaches have been adopted to protect against mycotoxicosis, however, additional studies using new environmentally friendly tools to combat mycotoxin contamination are still scarce and require further investigation.
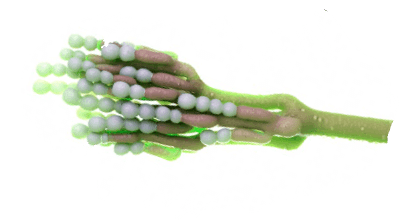

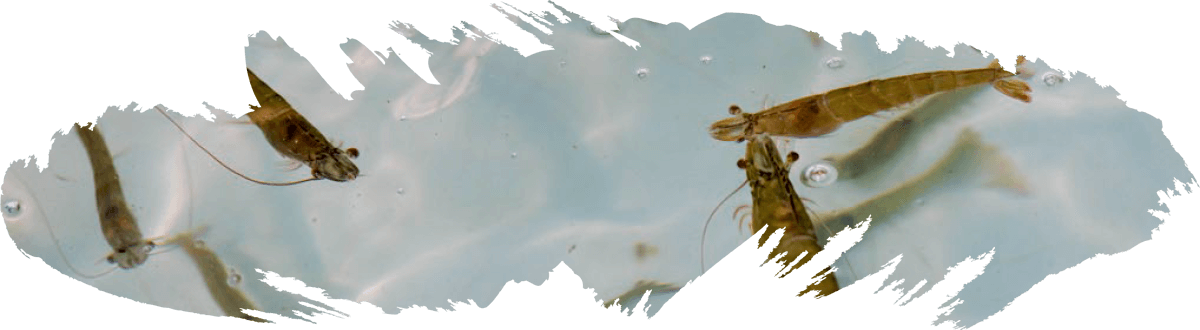
REFERENCES
Abrar, M., Anjum, F.M., Butt, M.S., Pasha, I., Randhawa, M.A., Saeed, F., Waqas, K., 2013. Aflatoxins: biosynthesis, occurrence, toxicity, and remedies. Critical reviews in food science and nutrition 53, 862 874.
Amoah, K., Huang, Q.-C., Tan, B.-P., Zhang, S., Chi, S.-Y., Yang, Q.-H., Liu, H.-Y., Dong, X.-H., 2019. Dietary supplementation of probiotic Bacillus coagulans ATCC 7050, improves the growth performance, intestinal morphology, microflora, immune response, and disease confrontation of Pacific white shrimp, Litopenaeus vannamei. Fish & shellfish immunology 87, 796-808.
Arunachalam, C., Doohan, F.M., 2013. Trichothecene toxicity in eukaryotes: Cellular and molecular mechanisms in plants and animals. Toxicology letters 217, 149-158.
Asim, M., Sarma, M.P., Thayumanavan, L., Kar, P., 2011. Role of aflatoxin B1 as a risk for primary liver cancer in north Indian population. Clinical biochemistry 44, 1235-1240.
Ayisi, C.L., Hua, X., Apraku, A., Afriyie, G., Kyei, B.A., 2017. Recent studies toward the development of practical diets for shrimp and their nutritional requirements. HAYATI Journal of Biosciences 24, 109-117.
Azad, M.A.K., Islam, S.S., Sithi, I.N., Ghosh, A.K., Banu, G.R., Bir, J., Huq, K.A., 2019. Effect of probiotics on immune competence of giant freshwater prawn Macrobrachium rosenbergii. Aquaculture Research 50, 644-657.
Baijal, P., Fitzpatrick, D., Bird, R., 1998. Modulation of colonic xenobiotic metabolizing enzymes by feeding bile acids: comparative effects of cholic, deoxycholic, lithocholic and ursodeoxycholic acids. Food and chemical toxicology 36, 601-607.
Bautista, M.N., Lavilla‐Pitogo, C.R., Subosa, P.F., Begino, E.T., 1994. Aflatoxin B1 contamination of shrimp feeds and its effect on growth and hepatopancreas of pre‐adult Penaeus monodon. Journal of the Science of Food and Agriculture 65, 5-11.
Bi, S., Huang, Z., Wang, Y., Nie, F., Wang, X., Sun, L., Zhu, Z., Gooneratne, R., 2019. Effects of T-2 toxin on histopathology, fatty acid and water distribution of shrimp (Litopenaeus vannamei) muscle. Journal of Environmental Science and Health, Part B 54, 416-423.
Bintvihok, A., Ponpornpisit, A., Tangtrongpiros, J., Panichkriangkrai, W., Rattanapanee, R., Doi, K., Kumagai, S., 2003. Aflatoxin contamination in shrimp feed and effects of aflatoxin addition to feed on shrimp production. Journal of food protection 66, 882-885.
Bosetti, C., Turati, F., La Vecchia, C., 2014. Hepatocellular carcinoma epidemiology. Best practice & research Clinical gastroenterology 28, 753-770.
Bulbul, M., Kader, M.A., Ambak, M.A., Hossain, M.S., Ishikawa, M., Koshio, S., 2015. Effects of crystalline amino acids, phytase and fish soluble supplements in improving nutritive values of high plant protein based diets for kuruma shrimp, Marsupenaeus japonicus. Aquaculture 438, 98-104.
Bulbul, M., Kader, M.A., Koshio, S., Ishikawa, M., Yokoyama, S., 2014. Effect of replacing fishmeal with canola meal on growth and nutrient utilization in kuruma shrimp M arsupenaeus japonicus (Bate). Aquaculture Research 45, 848-858.
Chen, S.-J., Gan, L., Guo, Y.-C., Tian, L.-X., Liu, Y.-J., 2020. Changes in growth performance, aflatoxin B1 residues, immune response and antioxidant status of Litopenaeus vannamei fed with AFB1-contaminated diets and the regulating effect of dietary myo-inositol supplementation. Food chemistry 324, 126888.
Čolović, R., Puvača, N., Cheli, F., Avantaggiato, G., Greco, D., Đuragić, O., Kos, J., Pinotti, L., 2019. Decontamination of mycotoxin-contaminated feedstuffs and compound feed. Toxins 11, 617.
Dayal, J.S., Rajaram, V., Ambasankar, K., Ali, S.A., 2011. Sunflower oil cake as a replacement for fish meal in feeds of Tiger Shrimp, Penaeus monodon reared in tanks and in net cages.
Deng, Y., Wang, Y., Zhang, X., Sun, L., Wu, C., Shi, Q., Wang, R., Sun, X., Bi, S., Gooneratne, R., 2017. Effects of T-2 Toxin on Pacific White Shrimp Litopenaeus vannamei: Growth, and Antioxidant Defenses and Capacity and Histopathology in the Hepatopancreas. Journal of Aquatic Animal Health 29, 15-25.
Dhanasekaran, D., Shanmugapriya, S., Thajuddin, N., Panneerselvam, A., 2011. Aflatoxins and aflatoxicosis in human and animals. Aflatoxins-Biochemistry and Molecular Biology 10, 221-254.
Diesing, A.-K., Nossol, C., Dänicke, S., Walk, N., Post, A., Kahlert, S., Rothkötter, H.-J., Kluess, J., 2011. Vulnerability of polarised intestinal porcine epithelial cells to mycotoxin deoxynivalenol depends on the route of application. PloS one 6, e17472.
Doi, K., Uetsuka, K., 2011. Mechanisms of mycotoxin-induced neurotoxicity through oxidative stress-associated pathways. International journal of molecular sciences 12, 5213-5237.
Duan, Y., Wang, Y., Dong, H., Ding, X., Liu, Q., Li, H., Zhang, J., Xiong, D., 2018. Changes in the intestine microbial, digestive, and immune-related genes of Litopenaeus vannamei in response to dietary probiotic Clostridium butyricum supplementation. Frontiers in Microbiology 9, 2191.
Fang, H., Wang, B., Jiang, K., Liu, M., Wang, L., 2020. Effects of Lactobacillus pentosus HC-2 on the growth performance, intestinal morphology, immune-related genes and intestinal microbiota of Penaeus vannamei affected by aflatoxin B1. Aquaculture 525, 735289.
Fegan, D., Spring, P. 2007. Recognizing the reality of the aquaculture mycotoxin problem: searching for a common and effective solution. In: Nutritional biotechnology in the feed and food industries: Proceedings of Alltech’s 23rd Annual Symposium. The new energy crisis: food, feed or fuel?, 343-354.
Ferlay, J., Shin, H.R., Bray, F., Forman, D., Mathers, C., Parkin, D.M., 2010. Estimates of worldwide burden of cancer in 2008: GLOBOCAN 2008. International journal of cancer 127, 2893-2917.
García‐Pérez, O.D., Tapia‐Salazar, M., Nieto‐López, M.G., Cruz‐Valdez, J.C., Maldonado-Muñiz, M., Guerrero Guerrero, L.M., Cruz‐ Suárez, L.E., Marroquín-Cardona, A.G., 2020. Effects of conjugated linoleic acid and curcumin on growth performance and oxidative stress enzymes in juvenile Pacific white shrimp (Litopenaeus vannamei) feed with aflatoxins. Aquaculture Research 51, 1051-1060.
Ghaednia, B., Bayat, M., Sohrabi Haghdoost, I., Motallebi, A., Sepahdari, A., Mirbakhsh, M., Mehrabi, M., 2013. Effects of aflatoxin B1 on growth performance, health indices, phagocytic activity and histopathological alteration in Fenneropenaeus indicus.
Gonçalves, R., Hofstetter, U., Schatzmayr, D., Jenkins, T., 2018a. Mycotoxins in Southeast Asian aquaculture: plant-based meals and finished feeds. World Mycotoxin Journal 11, 265-275.
Gonçalves, R., Schatzmayr, D., Hofstetter, U., Santos, G., 2017. Occurrence of mycotoxins in aquaculture: Preliminary overview of Asian and European plant ingredients and finished feeds. World Mycotoxin Journal 10, 183-194.
Gonçalves, R.A., Tarasco, M., Schatzmayr, D., Gavaia, P., 2018b. Preliminary evaluation of moniliformin as a potential threat for teleosts. Fishes 3, 4.
Gong, Y.Y., Wilson, S., Mwatha, J.K., Routledge, M.N., Castelino, J.M., Zhao, B., Kimani, G., Kariuki, H.C., Vennervald, B.J., Dunne, D.W., 2012. Aflatoxin exposure may contribute to chronic hepatomegaly in Kenyan school children. Environmental Health Perspectives 120, 893-896.
Grenier, B., Loureiro‐Bracarense, A.P., Leslie, J.F., Oswald, I.P., 2014. Physical and chemical methods for mycotoxin decontamination in maize. Mycotoxin reduction in grain chains, 116-129.
Huang, X., Huang, Z., Sun, L., Qiu, M., Deng, Q., Fang, Z., Wang, Y., 2022. Protective mechanisms of three antioxidants against T‐2 toxin‐induced muscle protein deterioration in shrimp. Journal of the Science of Food and Agriculture 102, 4883-4891.
Huang, Z., Sun, L., Wang, Y., Deng, Q., Fang, Z., Zhao, L., Zhao, J., 2021. Protective mechanism of tea polyphenols against muscle quality deterioration of shrimp (Penaeus vannamei) induced by aflatoxin B1. Aquaculture 532, 736093.
Huang, Z., Wang, Y., Qiu, M., Sun, L., Deng, Y., Wang, X., Bi, S., Gooneratne, R., Zhao, J., 2019. Effects of T-2 toxin on digestive enzyme activity, intestinal histopathology and growth in shrimp Litopenaeus vannamei. Scientific Reports 9, 13175.
Huang, Z., Wang, Y., Sun, L., Wang, X., Lu, P., Liang, G., Pang, H., Wu, Q., Gooneratne, R., Zhao, J., 2020. Effects of T‐2 toxin on the muscle proteins of shrimp (Litopenaeus vannamei)–a proteomics study. Journal of the Science of Food and Agriculture 100, 119-128.
Humans, I.W.G.o.t.E.o.C.R.t., 2014. DIESEL AND GASOLINE ENGINE EXHAUSTS AND SOME NITROARENES. IARC MONOGRAPHS ON THE EVALUATION OF CARCINOGENIC RISKS TO HUMANS. IARC monographs on the evaluation of carcinogenic risks to humans 105, 9.
Humans, I.W.G.o.t.E.o.C.R.t., Cancer, I.A.f.R.o., 2002. Some traditional herbal medicines, some mycotoxins, naphthalene and styrene, Vol 82. World Health Organization.
Ismaiel, A.A., Papenbrock, J., 2015. Mycotoxins: producing fungi and mechanisms of phytotoxicity. Agriculture 5, 492-537. Jamshidizadeh, S., Amrollahi Biuki, N., Yousefzadi, M., Aramideh, A., 2019. Response of Pacific white leg shrimp (Litopenaeus vannamei) on exposure to aflatoxin in feed. Aquaculture Research 50, 1973-1984.
Katya, K., Lee, S., Yun, H., Dagoberto, S., Browdy, C.L., Vazquez-Anon, M., Bai, S.C., 2016. Efficacy of inorganic and chelated trace minerals (Cu, Zn and Mn) premix sources in Pacific white shrimp, Litopenaeus vannamei (Boone) fed plant protein based diets. Aquaculture 459, 117-123.
Kew, M.C., 2013. Aflatoxins as a cause of hepatocellular carcinoma. Journal of Gastrointestinal & Liver Diseases 22. Macias-Sancho, J., Poersch, L.H., Bauer, W., Romano, L.A., Wasielesky, W., Tesser, M.B., 2014. Fishmeal substitution with Arthrospira (Spirulina platensis) in a practical diet for Litopenaeus vannamei: effects on growth and immunological parameters. Aquaculture 426, 120-125.
Marroquín-Cardona, A., Johnson, N., Phillips, T., Hayes, A., 2014. Mycotoxins in a changing global environment–a review. Food and Chemical Toxicology 69, 220-230.
Mexía-Salazar, A.L., Hernández-López, J., Burgos-Hernández, A., Cortez-Rocha, M.O., Castro-Longoria, R., Ezquerra-Brauer, J.M., 2008. Role of fumonisin B1 on the immune system, histopathology, and muscle proteins of white shrimp (Litopenaeus vannamei). Food chemistry 110, 471-479.
Minervini, F., Fornelli, F., Flynn, K., 2004. Toxicity and apoptosis induced by the mycotoxins nivalenol, deoxynivalenol and fumonisin B1 in a human erythroleukemia cell line. Toxicology in Vitro 18, 21-28.
Oliveira, M., Vasconcelos, V., 2020. Occurrence of mycotoxins in fish feed and its effects: A review. Toxins 12, 160.
Oujifard, A., Seyfabadi, J., Kenari, A.A., Rezaei, M., 2012. Growth and apparent digestibility of nutrients, fatty acids and amino acids in Pacific white shrimp, Litopenaeus vannamei, fed diets with rice protein concentrate as total and partial replacement of fish meal. Aquaculture 342, 56-61.
Pankaj, S., Shi, H., Keener, K.M., 2018. A review of novel physical and chemical decontamination technologies for aflatoxin in food. Trends in Food Science & Technology 71, 73-83.
Patriarca, A., Pinto, V.F., 2017. Prevalence of mycotoxins in foods and decontamination. Current Opinion in Food Science 14, 50-60.
Pérez-Acosta, J.A., Burgos-Hernandez, A., Velázquez-Contreras, C.A., Márquez-Ríos, E., Torres-Arreola, W., Arvizu-Flores, A.A., Ezquerra-Brauer, J.M., 2016. An in vitro study of alkaline phosphatase sensitivity to mixture of aflatoxin B 1 and fumonisin B 1 in the hepatopancreas of coastal lagoon wild and farmed shrimp Litopenaeus vannamei. Mycotoxin research 32, 117-125.
Pestka, J.J., 2010. Deoxynivalenol-induced proinflammatory gene expression: mechanisms and pathological sequelae. Toxins 2, 1300-1317.
Pleadin, J., Frece, J., Markov, K., 2019. Mycotoxins in food and feed. Advances in food and nutrition research 89, 297-345.
Qiu, M., Wang, Y., Wang, X., Sun, L., Ye, R., Xu, D., Dai, Z., Liu, Y., Bi, S., Yao, Y., 2016. Effects of T-2 toxin on growth, immune function and hepatopancreas microstructure of shrimp (Litopenaeus vannamei). Aquaculture 462, 35-39.
Radhika, G., Raj, R., George, K., Sanil, N., 2012. Ultrastructural changes in the hepatopancreas of Penaeus monodon Fabricius 1798 given aflatoxin B1 diets. Aquaculture Research 43, 32-43.
Reschly, E.J., Ai, N., Ekins, S., Welsh, W.J., Hagey, L.R., Hofmann, A.F., Krasowski, M.D., 2008. Evolution of the bile salt nuclear receptor FXR in vertebrates* s⃞. Journal of lipid research 49, 1577-1587.
Šarenac, T.M., Mikov, M., 2018. Bile acid synthesis: from nature to the chemical modification and synthesis and their applications as drugs and nutrients. Frontiers in Pharmacology 9, 939.
Sharawy, Z., Goda, A.M.-S., Hassaan, M.S., 2016. Partial or total replacement of fish meal by solid state fermented soybean meal with Saccharomyces cerevisiae in diets for Indian prawn shrimp, Fenneropenaeus indicus, Postlarvae. Animal Feed Science and Technology 212, 90-99.
Shu, X., Wang, Y., Zhou, Q., Li, M., Hu, H., Ma, Y., Chen, X., Ni, J., Zhao, W., Huang, S., 2018. Biological degradation of aflatoxin B1 by cell-free extracts of Bacillus velezensis DY3108 with broad pH stability and excellent thermostability. Toxins 10, 330.
Su, C., Li, J., Pan, L., Zhang, M., Chen, Z., Lu, M., 2023. Immunotoxicity and the mechanisms of aflatoxin B1-induced growth retardation in shrimp and alleviating effects of bile acids. Journal of Hazardous Materials 459, 132266.
Su, C., Lu, Y., Li, J., Wang, Y., Pan, L., Zhang, M., 2022. Effects of bile acids on aflatoxin B1 bioaccumulation, detoxification system, and growth performance of Pacific white shrimp. Food Chemistry 371, 131169.
Supamattaya, K., Sukrakanchana, N., Boonyaratpalin, M., Schatzmayr, D., Chittiwan, V., 2005. Effects of ochratoxin A and deoxynivalenol on growth performance and immuno-physiological parameters in black tiger shrimp (Penaeus monodon).
Songklanakarin Journal of Science and Technology 27, 91-99. Tacon, A.G., Jory, D., Nunes, A., 2013. Shrimp feed management: issues and perspectives. On-farm feeding and feed management in aquaculture 583, 481-488.
Tacon, A.G., Metian, M., 2008. Aquaculture feed and food safety: the role of the food and agriculture organization and the Codex Alimentarius. Annals of the New York Academy of Sciences 1140, 50-59.
Tapia-Salazar, M., García-Pérez, O.D., Nieto-López, M.G., Cruz-Valdez, J.C., Maldonado-Muñiz, M., Cruz-Suárez, L.E., Marroquín-Cardona, A.G., 2022. Growth parameters and activity of xenobiotic-metabolizing enzymes of juvenile Litopenaeus vannamei fed diets containing aflatoxins and an aflatoxin binder. Hidrobiológica 32, 141-148.
Tapia-Salazar, M., García-Pérez, O.D., Velásquez-Soto, R.A., Nieto-López, M.G., Villarreal-Cavazos, D., Ricque-Marie, D., Cruz-Suárez, L.E., 2012. Growth, feed intake, survival, and histological response of white shrimp Litopenaeus vannamei fed diets containing grains naturally contaminated with aflatoxin. Ciencias Marinas 38, 491 504.
Varga, J., Frisvad, J.C., Samson, R., 2011. Two new aflatoxin producing species, and an overview of Aspergillus section Flavi. Studies in mycology 69, 57-80.
Vila-Donat, P., Marín, S., Sanchis, V., Ramos, A., 2018. A review of the mycotoxin adsorbing agents, with an emphasis on their multi-binding capacity, for animal feed decontamination. Food and chemical toxicology 114, 246-259.
Wang, A., Ran, C., Wang, Y., Zhang, Z., Ding, Q., Yang, Y., Olsen, R.E., Ringø, E., Bindelle, J., Zhou, Z., 2019. Use of probiotics in aquaculture of China—a review of the past decade. Fish & shellfish immunology 86, 734-755.
Wang, J., Guo, R., Su, L., Xia, H., Cui, M., 2012. Toxic effects of aflatoxin B1 on growth performance, biochemical and hepatopancreas microstructure of Litopanaeus vannamei. Journal of Fisheries of China 36, 952-957.
Wang, Y., Wang, B., Liu, M., Jiang, K., Wang, M., Wang, L., 2018. Aflatoxin B1 (AFB1) induced dysregulation of intestinal microbiota and damage of antioxidant system in pacific white shrimp (Litopenaeus vannamei). Aquaculture 495, 940-947.
Wu, Q.-H., Wang, X., Yang, W., Nüssler, A.K., Xiong, L.-Y., Kuča, K., Dohnal, V., Zhang, X.-J., Yuan, Z.-H., 2014. Oxidative stress-mediated cytotoxicity and metabolism of T-2 toxin and deoxynivalenol in animals and humans: an update. Archives of toxicology 88, 1309-1326.
Xie, S., Zheng, L., Wan, M., Niu, J., Liu, Y., Tian, L., 2018. Effect of deoxynivalenol on growth performance, histological morphology, anti-oxidative ability and immune response of juvenile Pacific white shrimp, Litopenaeus vannamei. Fish & shellfish immunology 82, 442-452.
Yang, Q., Tan, B., Dong, X., Chi, S., Liu, H., 2015. Effect of replacing fish meal with extruded soybean meal on growth, feed utilization and apparent nutrient digestibility of juvenile white shrimp (Litopenaeus vannamei). Journal of Ocean University of China 14, 865-872.
Yang, X., Lv, Y., Huang, K., Luo, Y., Xu, W., 2016. Zinc inhibits aflatoxin B1-induced cytotoxicity and genotoxicity in human hepatocytes (HepG2 cells). Food and Chemical Toxicology 92, 17-25.
Yu, Y.-Y., Niu, J., Yin, P., Mei, X.-T., Liu, Y.-J., Tian, L.-X., Xu, D.-H., 2018. Detoxification and immunoprotection of Zn(II)-curcumin in juvenile Pacific white shrimp (Litopenaeus vannamei) feed with aflatoxin B1. Fish & Shellfish Immunology 80, 480-486.
Zeng, S., Long, W., Tian, L., Xie, S., Chen, Y., Yang, H., Liang, G., Liu, Y., 2016. Effects of dietary aflatoxin B1 on growth performance, body composition, haematological parameters and histopathology of juvenile Pacific white shrimp (Litopenaeus vannamei). Aquaculture Nutrition 22, 1152-1159.
Zhang, C., Zhang, J., Fan, W., Huang, M., Liu, M., 2019. Effects of dietary Lactobacillus delbrueckii on growth performance, body composition, digestive and absorptive capacity, and gene expression of common carp (Cyprinus carpio Huanghe var). Aquaculture Nutrition 25, 166-175.
Zhao, W., Wang, L., Liu, M., Jiang, K., Wang, M., Yang, G., Qi, C., Wang, B., 2017. Transcriptome, antioxidant enzyme activity and histopathology analysis of hepatopancreas from the white shrimp Litopenaeus vannamei fed with aflatoxin B1 (AFB1). Developmental & Comparative Immunology 74, 69-81.
Zuo, Z.-h., Shang, B.-j., Shao, Y.-c., Li, W.-y., Sun, J.-s., 2019. Screening of intestinal probiotics and the effects of feeding probiotics on the growth, immune, digestive enzyme activity and intestinal flora of Litopenaeus vannamei. Fish & shellfish immunology 86, 160-168.