Mursal Abdulkadir Hersi1, Dogukan Kaya2 and Ercument Genc1*
1Department of Fisheries and Aquaculture Engineering, Faculty of Agriculture, Ankara University, Ankara, Turkey
2Agricultural Applications and Research Center, Tokat Gaziosmanpasa University, Tokat, Turkey
Corresponding author: [email protected]
Mycotoxins are toxic and potentially carcinogenic substances produced by different types of fungi. Mycotoxin-induced diseases have severe economic consequences for the aquaculture industry.
So far, different methods of both chemical, microbiological and physical mechanisms that can either eliminate or reduce the effect of mycotoxins are available.

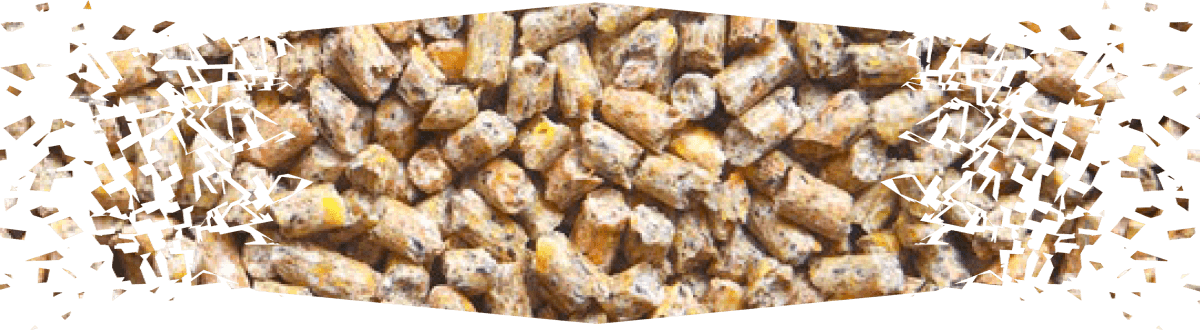
Micotoxins risk in Aquafeed
The world food supply system is expected to be under massive pressure by the end of 2050, when the human population is projected to reach up to 10 billion (Millington and Cleland, 2017). This increasing human population will represent a tremendous demand for animal protein and fish products (Tilman and Clark, 2014).
The fishing industry has also led to the depletion of wild aquatic animals.
Capture fisheries are no longer seen as a sustainable source for supplying our ever-increasing protein demand. Therefore, aquaculture is considered the best candidate to meet our animal protein needs (Subasinghe et al., 2009).
With the expansion of the aquaculture sector, the demand for aquafeed, which is an integral part of the production cost accounting for approximately 60% of the total fish production cost in intensive and super-intensive farming systems, is increasing (Alceste y Jory, 2000).
Mainstream fish farmers in tropical and developing regions use home-produced instead of imported fish feed because imported feed is usually expensive.
To manufacture local feed pellets, the farmers utilize animal waste and local plants such as soybeans, maize, rice, and many other grains (Liti et al. 2005). Since no vital biosecurity measurements are taken during the feed formulation process, such feeds are usually susceptible to contamination with moulds before and after storage (Almeida et al., 2012) and some of them can produce mycotoxins.
Mycotoxins are toxic and potentially carcinogenic substances produced by different types of fungi (Zain et al., 2012).
The formation and concentration of mycotoxins in aquaculture are primarily affected by temperature and humidity. Still, many other elements also affect the growth of moulds, such as:
Water movement
Substrates
pH levels
Feed storage conditions
Microbial abundance and interactions
(Di Gregorio et al., 2014)
Infections triggered by mycotoxins are known as mycotoxicosis and are influenced by the dose, species, age, and duration of intoxication.
Depending on the type of mycotoxin, different pathological symptoms can be observed in the affected species, such as anaemia, liver and kidney lesions, hemorrhaging, increased susceptibility to other diseases, and even death (Jaynes et al., 2007).
Mycotoxin-induced diseases cause severe economic reductions in the aquaculture industry (Di Gregorio et al. 2014).
For this reason, in recent years, researchers have made an effort to examine possible strategies for decontamination of mycotoxins in aquaculture (Bovo et al., 2012). To prevent the occurrence of mycotoxins, strong biosecurity measures before and after aquafeed storage are recommended (Di Gregorio et al. 2014). However, the occurrence of mycotoxins is challenging to avoid (Kendra and Dyer, 2007).
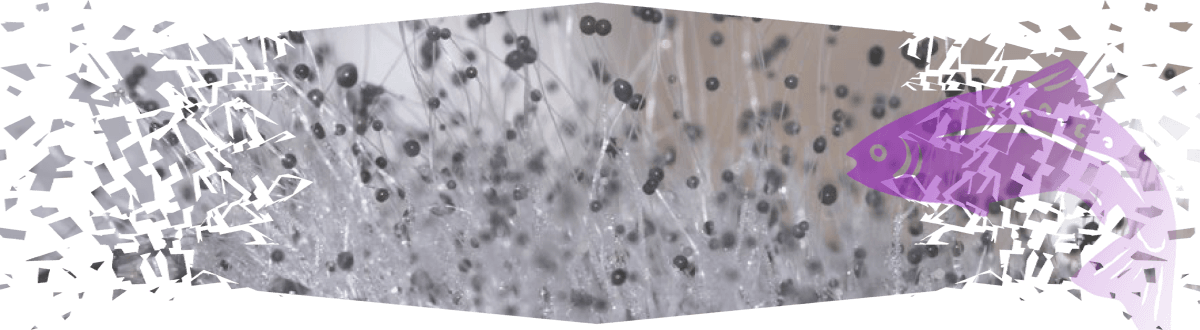
The occurrence and toxicity of mycotoxins in aquaculture
Studies on the negative impact of mycotoxins in animals have focused mainly on terrestrial livestock rather than aquatic farmed animals (Pestka, 2007). It was not until the outbreak of aflatoxins in the rainbow trout industry in the 1960s that researchers started to make an effort to study the effects of mycotoxins in aquaculture (Gonçalves et al., 2018).
Due to the higher cost of aquaculture feed ingredients and fish oil, in particular, researchers have sought to identify potential protein source alternatives. Plant proteins can serve as an excellent substitute for fish oil in aquatic feeds, as they are cost-effective and available on a large scale (Anater et al. 2016).
Currently, plant proteins such as oilseeds and grains are included in most aquatic feeds formulated for warm water finfish such as tilapia, carp, and catfish (Marijani et al., 2019).
This type of feed is very vulnerable to fungal contamination, so the occurrence of mycotoxins is inevitable (Marijani et al., 2017).
According to Berthiller et al. (2007), over 400 mycotoxin types are known worldwide and, among them, aflatoxins are the best known due to their frequency of incidence, followed by ochratoxins and Fusarium mycotoxins. Apart from these main mycotoxins, others with low frequency of occurrences, such as nivalenol, alternariol, and roquefortine C, are detected in fish feed (Marijani et al., 2017).
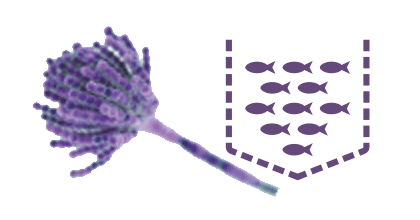
Table 1. Main mycotoxins and their associated toxicity in aquaculture.
Aflatoxins
Aflatoxins are water-soluble substances produced by moulds from the genus Aspergillus, particularly Aspergillus parasiticus and Aspergillus flavus (Varga et al., 2009).
There are approximately 20 aflatoxins detected worldwide, but only four of them (AFB1, AFB2, AFG1 and AFG2) have been studied much more than others simply because they are the most toxic aflatoxin molecules (Santacroce et al., 2008).
A. parasiticus can produce all of the earlier mentioned compounds, whereas A. flavus can only produce aflatoxins B1 and B2. However, both species can contaminate pre- and post-storage conditions of aquafeeds (Bryden, 2012).
Aflatoxins mainly occur in humid climates but they have also been reported in tropical and subtropical regions (Ostrowski-Meissner et al. 1995; Marin et al. 2013).
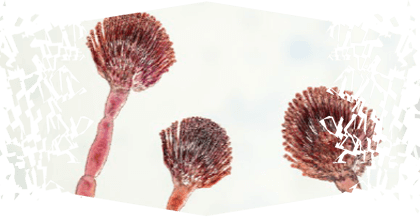
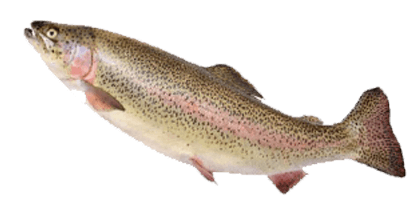
A study conducted by Alinezhad et al. (2011), investigated the presence of aflatoxins in the ingredients and feed formulated for rainbow trout.

The biological effects of aflatoxins are hazardous. The most potent aflatoxin type is AFB1 which can:
Cause severe liver damage
Promote cancer
Induce genetic mutations
Halt the reproduction process
Substantially weaken the immune system
Aflatoxin-related toxicity depends on the concentration, life stage, and species of the farmed animal (Hendricks, 1993).
In aquaculture, aflatoxins were first identified in 1963 during a hepatoma epizootic in the trout industry when fish were fed aflatoxin-contaminated feed (Ashley and Halver, 1963). Similar aflatoxin poisoning events in different fish species have been reported since then (Ashley et al., 1965; Cagauan et al., 2004; Zhang et al., 2022).
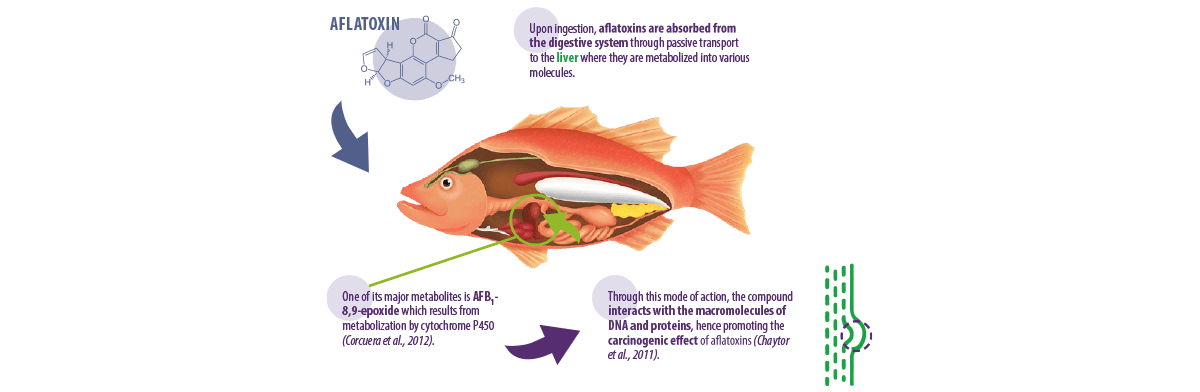
There are three stages of aflatoxicosis: acute, subacute, and chronic.
ACUTE AFLATOXICOSIS
The acute phase occurs when an aquatic animal consumes a moderate or elevated dose of aflatoxins in a short time, resulting in health consequences such as;
Anaemia
Whitish or pale gills
Haemorrhaging
Poor nutrient utilization
Hepatotoxicity
(Matejova et al., 2017a)
A 10-day experiment conducted by Sahoo et al. (2001) with carp revealed that the ingestion of aflatoxin B1 by Labeo rohita at a dose of 11.25 mg/kg caused significantly reduced appetite, lethargy, and loss of balance.
When tissue histomorphology was analysed, they also found seriously damaged kidneys and gills, necrotic liver with vascular dilatation and congestion, as well as sloughed intestines.
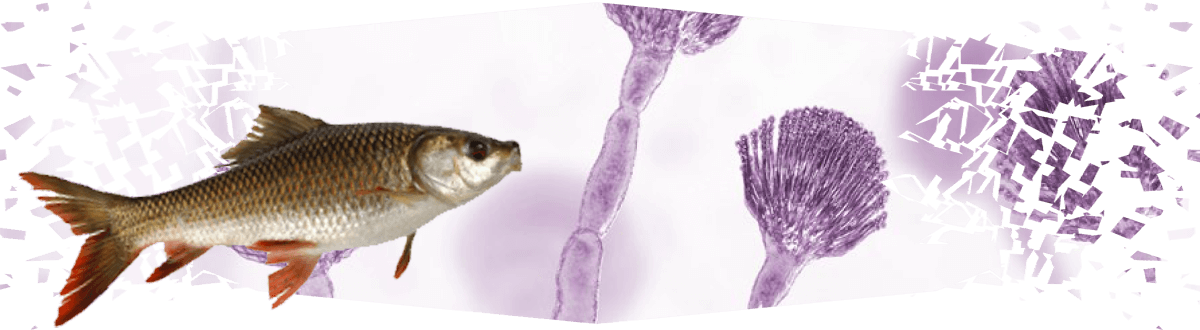
Subacute and chronic forms occur when animals are exposed to low doses of aflatoxin over a relatively long period, typically about three and six months, respectively.
SUBACUTE AFLATOXICOSIS
Pathological symptoms of subacute aflatoxicosis include:
Immunosuppression
Hepatotoxicity
Yellowish skin
Failed nutrient adsorption
(Santacroce et al., 2008)
Symptoms such as liver necrosis, vascular dilation, occlusion, and significant reduction of immune response and antioxidant enzymes were reported in the study by Sahoo et al. (2001) when a 2.5 mg/kg dose of aflatoxin B1 was given to Labeo rohita for 90 days.
CHRONIC AFLATOXICOSIS
The chronic stage of aflatoxin exposure is associated with:
Carcinogenicity
Immunosuppression
Hepatotoxicity
Mutagenicity
Haematological alterations
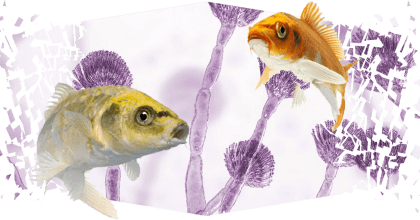
The main chronic histopathological symptoms observed in different fish species include liver failure, cessation of feed intake, weakened immune system, and death (Santacroce et al., 2008).
Moreover, a study conducted by Shahafve et al. (2017) evaluated the effects of diets containing different doses of aflatoxins (0.5, 0.7, and 1.4 mg/ kg) on common carp (Cyprinus carpio) tissue histomorphology for 3 weeks.
Pathological examinations revealed irregular and shrunken gill lamellae, necrotic and swollen liver hepatocytes, vascular congestion, damaged liver parenchyma, renal urinary space dilation, abnormal increase in goblet cells, sloughed intestines, and inflammation.
Fusarium mycotoxins
Fusarium moulds produce a variety of potent mycotoxins, but three of them have so far undergone substantial research studies (Placinta et al., 2016):
- ⇰ Trichothecenes
- ⇰ Fumonisins
- ⇰ Zearalenone
Globally, many reports show the incidence of Fusarium mycotoxin in grains and aquatic animal feed, especially when they are under humid conditions (Pinotti et al., 2016; Bryden, 2012).
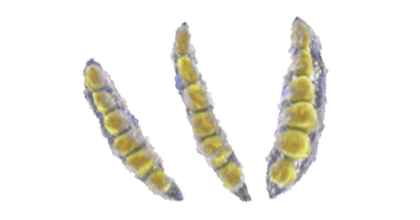
TRICHOTHECENES
Trichothecenes are mycotoxins produced by fungi species belonging to different genera such as Fusarium, Myrothecium, and Trichothecium (Tamm & Breitenstein, 1984). There are four well-known trichothecenes:
- ⇰ Deoxynivalenol (DON)
- ⇰ T-2 toxin
- ⇰ Diacetoxyscirpenol (DAS)
- ⇰ Nivalenol (NIV)
(Escrivá et al., 2015)
These compounds contaminate cereals, for instance, maize, soybeans, wheat, and similar grains, all of which are significant components of aquafeeds (Meronuck and Xie, 1999).
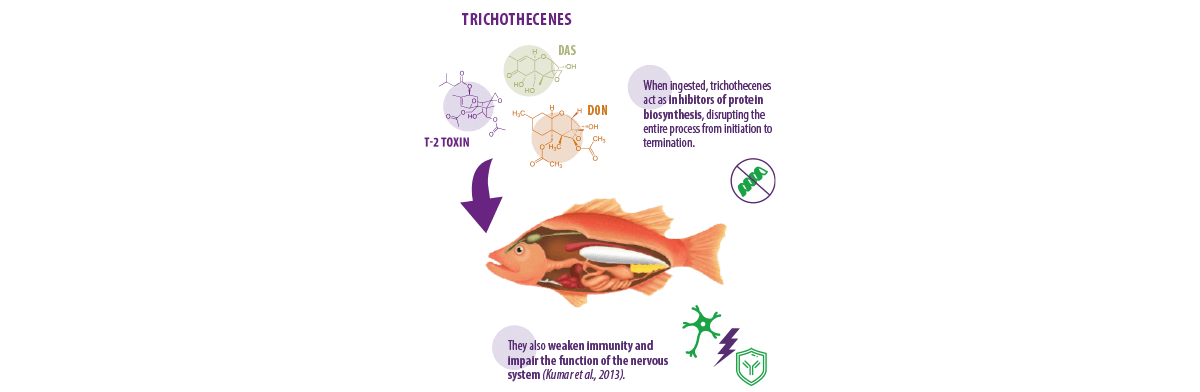
Animals exposed to trichothecenes for a long time show various clinical signs, such as:
Cessation of feeding
Sluggish movement
Growth restriction
Tissue lesions
Higher doses of this mycotoxin also trigger shock-like syndrome, eventually resulting in mortality (Matejova et al., 2017a).

T-2 TOXIN
An experiment carried out by Poston et al. (1982) assessed the adverse effects of higher and lower doses (2.5 and 15 mg/kg) of T-2 toxin on trout (Oncorhynchus mykiss).
The results indicated reduced growth rate and feed utilization parameters in the groups ingested lower toxic levels, while those exposed to higher doses showed sloughed intestines, haemorrhaging, and significantly damaged spleens.
Another study carried out by Manning et al. (2003) examined the biological effect of four different T-2 toxin dosages (0.63, 1.3, 2.5, & 5 mg/kg) on the growth and tissue histomorphology of channel catfish (Ictalurus punctatus).
Significantly reduced growth rate and feed utilization indexes, as well as lesions in the intestine and liver, were reported in this study.
A more recent study by Matejova et al. (2017) evaluated the effect of 5.3 mg/kg T-2 toxin in diet on the growth, immune and haematological indices of common carp (Cyprinus carpio) for 30 days.
Negatively affected haematological parameters such as haemoglobin, red and white blood cells, and reduced antioxidant enzymes, as well as tissue lesions, were noted in this study.
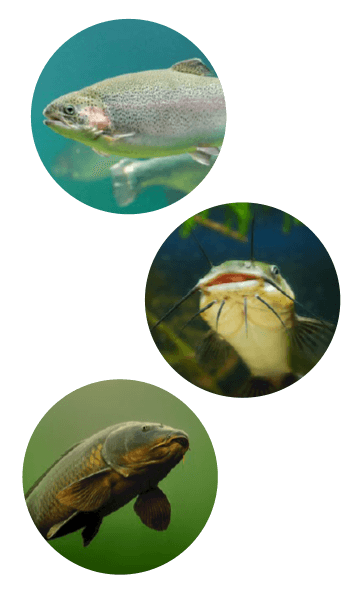
DON
Deoxynivalenol, also called vomitoxin, is generally less toxic but more common than T-2 toxin. Like other trichothecenes, it occurs in grains, particularly wheat and maize, contaminating aquafeed containing any of these grains (Marin et al., 2013).
Pathological signs of vomitoxin ingestion aquaculture species include:
Growth retardation
Degenerated and sloughed intestine
Inflammation
Poor nutrient utilization
Immunosuppression
(Hooft et al., 2011)
Huang et al. (2020) evaluated how deoxynivalenol at five different doses (0.03, 0.32, 0.63, 0.92 and 1.24 mg/kg) affected the gill histomorphology of grass carp (Ctenopharyngodan idella) over 2 months.
The results of this study depicted a considerable reduction in the antioxidant capacity of the fish, as well as lesions and injury of both the gills and their tight junctions.
FUMONISINS
Fumonisins are mainly produced by Fusarium verticillioides and F. proliferatum, which are species that are frequently found contaminating maize. Among the different types of this mycotoxin, the most well-known is Fumonisin B1 (Scott, 2012).
In aquaculture, fumonisins are linked to:
Growth retardation
Poor nutrient utilization
Halted sphingolipid metabolism
(Tuan et al., 2003)
Fumonisins have a long hydrocarbon chain resembling sphingosine, an enzyme that helps catalyze the alkylation and diacylation of sphingolipids during lipid metabolism.
⇰ Fumonisin B1, therefore, acts as an inhibitor of sphingolipid synthesis which is crucial for membrane and lipoprotein structures (Wang et al., 1992).
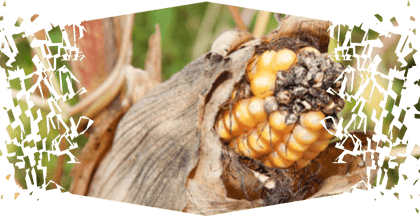
Claudino-Silva et al. (2018) examined the toxicity of diets containing Fumonisin B1 and B2 at various doses (20, 40, and 60 mg/kg) on the growth and feed utilization of tilapia (Oreochromis niloticus).
At the end of this trial, significant growth and growth hormone reduction and poor nutrient utilization were detected.
ZEARALENONE
Zearalenone is another Fusarium mycotoxin mainly produced by Fusarium culmorum and Fusarium graminearum, appearing in different grains such as maize and wheat (Henderson y Smith, 1991).
The production of ZEN is associated with high moisture and lower temperature conditions, and it is linked to reproductive alterations in the exposed animals (Placinta et al., 1999).
⇰ The metabolites produced by zearalenone are structurally similar to natural estrogens and, by mimicking these hormones, they bind with estrogen receptors halting the reproduction of the animal (Kumar et al., 2013).
A three-week experiment conducted by Schwartz et al. (2010) investigating the effects of zearalenone on zebrafish (Danio rerio) reproduction found that it reduced both the fish’s fertility and spawning frequency. Similar results were reported in a study conducted by Woźny et al. (2015) with rainbow trout.
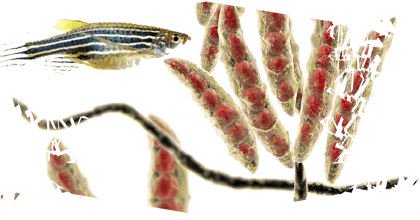
OCHRATOXINS
Ochratoxins are toxic secondary metabolites produced by fungi belonging to the genera Aspergillus and Penicillium. Among the Ochratoxin groups, ochratoxin A (OTA) is the most prevalent and is known for its toxicity for kidney and liver in domesticated animals (Manning and Wyatt, 1984) and residues in the exposed animal’s meat (Guillamont et al., 2005).
⇰ Significantly lower feed utilization rate, growth retardation, premature mortality, and necrotic kidney and liver are among the clinical signs reported in aquaculture species exposed to OTA (Manning et al., 2003).
A study carried out by Hagelberg et al. (1989) assessed how three different doses of OTA (4, 6, and 8 mg/kg) affected the liver and kidney histomorphology of rainbow trout.
Results depicted that the groups fed diets containing 4 mg/kg OTA had moderate kidney and liver damage while those with 8 mg/kg showed severe hepatotoxicity and nephrotoxicity.
Similarly, El-Sayed et al. (2009) examined the sensitivity and clinical symptoms of sea bass (Dicentrarchus labrax) fed with diets supplemented with OTA at a dose of 9.23 mg/kg.
Lethargy, loss of balance, and haemorrhaging dorsal and eroded ventral surfaces were among the observed clinical signs before death. At the same time, necropsy revealed necrotic liver with severe vascular dilatation and congestion, as well as damaged kidneys.
A more recent study by Baldissera et al. (2020) investigated the effects of OTA (2.4 mg/kg in feed) on the growth, feed utilization, and antioxidant enzymes of tambaqui (Colossoma macropomum).
Significant reduction of growth and feed utilization indices, as well as higher antioxidant enzymes in fish muscles, were reported in this study.
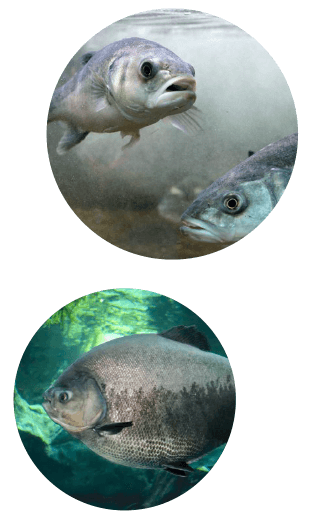
Table 2. Summary of recent studies on the effect of mycotoxins in different aquatic animals. Dose, duration, and toxicity are reviewed.

REFERENCES
Adeyemo, B.T., Tiamiyu, L.O., Ayuba, V.O. and Cheikyula, J.O., 2016. Effects of dietary fumonisin B1 on hematology and growth performance of the clariid fish Heterobranchus longifilis. J. Agric. Vet. Sci, 9, 26-33.
Adeyemo, B.T., Tiamiyu, L.O., Ayuba, V.O., Musa, S. and Odo, J., 2018. Effects of dietary mixed aflatoxin B1 and fumonisin B1 on growth performance and hematology of juvenile Clarias gariepinus catfish. Aquaculture, 491, 190-196.
Alceste, C. and Jory, D.E., 2000. Tilapia alternative protein sources in tilapia feed formulation. Aquaculture Magazine-Arkansas, 26(4), 70-75.
Alinezhad, S., Tolouee, M., Kamalzadeh, A., Motalebi, A.A., Nazeri, M., Yasemi, M., Shams, G.M., Tolouei, R. and Razzaghi, A.M., 2011. Mycobiota and aflatoxin B1 contamination of rainbow trout (Oncorhynchus mykiss) feed with emphasis to Aspergillus section Flavi. Iranian Journal of Fisheries, 10(3), 363-374.
Almeida, I.F.M., Martins, H.M.L., Santos, S.M.O., Freitas, M.S., da Costa, J.M.G.N. and d´ Almeida Bernardo, F.M., 2011. Mycobiota and aflatoxin B1 in feed for farmed sea bass (Dicentrarchus labrax). Toxins, 3(3), 163-171.
Anater, A., Manyes, L., Meca, G., Ferrer, E., Luciano, F.B., Pimpao, C.T. and Font, G., 2016. Mycotoxins and their consequences in aquaculture: A review. Aquaculture, 451, 1-10.
Ashley, L.M. and Halver, J.E., 1963. Multiple metastasis of rainbow trout hepatoma. Transactions of the American Fisheries Society, 92(4), 365-371.
Ashley, L.M., Halver, J.E., Gardner, W.K. and Wogan, G.N., 1965, January. Crystalline aflatoxins cause trout hepatoma. In Federation proceedings (9650 Rockville Pike, Bethesda, MD 20814-3998: Federation Amer. Soc. Exp. Biol. 24 (2),627
Baldissera, M.D., Souza, C.F., da Silva, J.A., Barroso, D.C., Glória, E.M., Mesadri, J., Wagner, R., Baldisserotto, B. and Val, A.L., 2020. Dietary ochratoxin A (OTA) decreases growth performance and impairs the muscle antioxidant system and meat fatty acid profiles in juvenile tambaqui (Colossoma macropomum). Comparative Biochemistry and Physiology Part C: Toxicology & Pharmacology, 236, 108803.
Baldissera, M.D., Souza, C.F., Zeppenfeld, C.C., Descovi, S.N., Moreira, K.L.S., da Rocha, M.I.U., da Veiga, M.L., da Silva, A.S. and Baldisserotto, B., 2018. Aflatoxin B1-contaminated diet disrupts the blood–brain barrier and affects fish behavior: involvement of neurotransmitters in brain synaptosomes. Environmental Toxicology and Pharmacology, 60, 45-51.
Bovo F, Corassin CH, Rosim RE, Oliveira CAF. (2012). Efficiency of actic acid bacteria strains for decontamination of aflatoxin M1 in phosphate buffer saline solution and in skimmed milk. Food Bioprocess Tech, 5:1-5
Bryden, W.L., 2012. Mycotoxin contamination of the feed supply chain: Implications for animal productivity and feed security. Animal Feed Science and Technology, 173(1-2), 134-158.
Cagauan, A.G., Tayaban, R.H., Somga, J.R. and Bartolome, R.M., 2004, September. Effect of aflatoxin contaminated feeds in Nile tilapia (Oreochromis niloticus L.). In: Abstract of the 6th International Symposium on Tilapia in Aquaculture (ISTA 6) Section: Health Management and Diseases, Manila, Philippines (Vol. 12, pp. 16).
Chaytor, A.C., Hansen, J.A., Van Heugten, E., See, M.T. and Kim, S.W., 2011. Occurrence and decontamination of mycotoxins in swine feed. Asian-Australasian Journal of Animal Sciences, 24(5), 723-738.
Claudino-Silva, S.C., Lala, B., Mora, N.H.A.P., Schamber, C.R., Nascimento, C.S., Pereira, V.V., Hedler, D.L. and Gasparino, E., 2018. Challenge with fumonisins B1 and B2 changes IGF-1 and GHR mRNA expression in liver of Nile tilapia fingerlings. World Mycotoxin Journal, 11(2), 237-245.
Corcuera, L.A., Vettorazzi, A., Arbillaga, L., Gonzalez-Peñas, E. and De Cerain, A.L., 2012. An approach to the toxicity and toxicokinetics of aflatoxin B1 and ochratoxin A after simultaneous oral administration to fasted F344 rats. Food and Chemical Toxicology, 50(10), 3440-3446.
Di Gregorio, M.C., Neeff, D.V.D., Jager, A.V., Corassin, C.H., Carão, Á.C.D.P., Albuquerque, R.D., Azevedo, A.C.D. and Oliveira, C.A.F., 2014. Mineral adsorbents for prevention of mycotoxins in animal feeds. Toxin Reviews, 33(3), 125-135.
El-Sayed, Y.S., Khalil, R.H. and Saad, T.T., 2009. Acute toxicity of ochratoxin-A in marine water-reared sea bass (Dicentrarchus labrax L.). Chemosphere, 75(7), 878-882.
Escrivá, L., Font, G. and Manyes, L., 2015. In vivo toxicity studies of fusarium mycotoxins in the last decade: A review. Food and Chemical Toxicology, 78, 185-206.
Berthiller, F. Sulyok, M.Krska, R. and Schuhmacher, R., 2007. Chromatographic methods for the simultaneous determination of mycotoxins and their conjugates in cereals, International Journal of Food Microbiology, 119(1-2), 33–37.
Ghafarifarsani, H., Imani, A., Niewold, T.A., Pietsch-Schmied, C. and Moghanlou, K.S., 2021. Synergistic toxicity of dietary aflatoxin B1 (AFB1) and zearalenone (ZEN) in rainbow trout (Oncorhynchus mykiss) is attenuated by anabolic effects. Aquaculture, 541, 736793.
Gonçalves, R.A., Naehrer, K. and Santos, G.A., 2018. Occurrence of mycotoxins in commercial aquafeeds in Asia and Europe: a real risk to aquaculture? Reviews in Aquaculture, 10(2), 263-280.
Guillamont, E.M., Lino, C.M., Baeta, M.L., Pena, A.S., Silveira, M.I.N. and Vinuesa, J.M., 2005. A comparative study of extraction apparatus in HPLC analysis of ochratoxin A in muscle. Analytical and Bioanalytical Chemistry, 383(4), 570-575.
Hagelberg, S., K. Hult, and R. Fuchs. 1989. Toxicokinetics of ochratoxin A in several species and its plasma binding properties. Journal of Applied Toxicology, 9:91–96.
Henderson, R.S. and Smith, J.E. eds., 1991. Mycotoxins and animal foods. CRC Press.
Hendricks, J.D., 1993. Carcinogenicity of aflatoxins in nonmammalian organisms. In: The toxicology of aflatoxins: human health, veterinary and agricultural significance. Eds: Eaton, D. L.; Groopman, J. D., 103 136. Academic Press, San Diego, USA.
Hooft, J.M., Encarnação, P. and Bureau, D.P., 2011. Rainbow trout (Oncorhynchus mykiss) is extremely sensitive to the feed-borne Fusarium mycotoxin deoxynivalenol (DON). Aquaculture, 311(1-4), 224-232.
Huang, C., Feng, L., Liu, X.A., Jiang, W.D., Wu, P., Liu, Y., Jiang, J., Kuang, S.Y., Tang, L. and Zhou, X.Q., 2020. The toxic effects and potential mechanisms of deoxynivalenol on the structural integrity of fish gill: Oxidative damage, apoptosis and tight junctions disruption. Toxicon, 174, 32-42.
Huang, C., Wu, P., Jiang, W.D., Liu, Y., Zeng, Y.Y., Jiang, J., Kuang, S.Y., Tang, L., Zhang, Y.A., Zhou, X.Q. and Feng, L., 2018a. Deoxynivalenol decreased the growth performance and impaired intestinal physical barrier in juvenile grass carp (Ctenopharyngodon idella). Fish & Shellfish Immunology, 80, 376-391.
Jaynes, W.F., Zartman, R.E. and Hudnall, W.H., 2007. Aflatoxin B1 adsorption by clays from water and corn meal. Applied Clay Science, 36(1-3), 197-205.
Kendra, D.F. and Dyer, R.B., 2007. Opportunities for biotechnology and policy regarding mycotoxin issues in international trade. International Journal of Food Microbiology, 119(1-2), 147-151.
Kumar, V., Roy, S., Barman, D., Kumar, A., Paul, L. and Meetei, W.A., 2013. Importance of mycotoxins in aquaculture feeds. Aquaculture Asia, 18(1), 25-29.
Liti, D., Cherop, L., Munguti, J. and Chhorn, L., 2005. Growth and economic performance of Nile tilapia (Oreochromis niloticus L.) fed on two formulated diets and two locally available feeds in fertilized ponds. Aquaculture Research, 36(8), 746-752.
Liu, X., Wu, P., Jiang, W.D., Liu, Y., Jiang, J., Kuang, S.Y., Tang, L., Zhou, X.Q. and Feng, L., 2020. Effects of dietary ochratoxin a on growth performance and intestinal apical junctional complex of juvenile grass carp (Ctenopharyngodon idella). Toxins, 13(1), 11.
Mahfouz, M.E. and Sherif, A.H., 2015. A multiparameter investigation into adverse effects of aflatoxin on Oreochromis niloticus health status. The Journal of Basic & Applied Zoology, 71, 48-59.
Manning, B. B., R. M. Ulloa, M. H. Li, E. H. Robinson, and G. E. Rottinghaus. 2003. Ochratoxin A fed to channel catfish (Ictalurus punctatus) causes reduced growth and lesions of hepatopancreatic tissue. Aquaculture, 219:739–750.
Manning, R.O. and Wyatt, R.D., 1984. Effect of cold acclimation of broiler chicks on susceptibility to acute aflatoxicosis. Poultry Science, 63, p.24S.
Marijani, E., Charo-Karisa, H., Gnonlonfin, G.J.B., Kigadye, E. and Okoth, S., 2019. Effects of aflatoxin B1 on reproductive performance of farmed Nile tilapia. International Journal of Veterinary Science and Medicine, 7(1), 35-42.
Marijani, E., Wainaina, J.M., Charo-Karisa, H., Nzayisenga, L., Munguti, J., Gnonlonfin, G.J.B., Kigadye, E. and Okoth, S., 2017. Mycoflora and mycotoxins in finished fish feed and feed ingredients from smallholder farms in East Africa. The Egyptian Journal of Aquatic Research, 43(2), 169-176.
Marin, S., A. J. Ramos, G. Cano-Sancho, and V. Sanchis. 2013. Mycotoxins: occurrence, toxicology, and exposure assessment. Food and Chemical Toxicology, 60: 218–237.
Matejova, I., Faldyna, M., Modra, H., Blahova, J., Palikova, M., Markova, Z., Franc, A., Vicenova, M., Vojtek, L., Bartonkova, J. and Sehonova, P., 2017b. Effect of T-2 toxin-contaminated diet on common carp (Cyprinus carpio L.). Fish & Shellfish Immunology, 60, 458-465.
Matejova, I., Svobodova, Z., Vakula, J., Mares, J. and Modra, H., 2017a. Impact of mycotoxins on aquaculture fish species: a Review. Journal of the World Aquaculture Society, 48(2), 186-200.
Meronuck, R.A., 1994. Mycotoxins in feed. Feedstuffs (USA). Millington, K.A. and Cleland, J. 2017. Counting people and making people count: Key sources of population projections, K4D Helpdesk Report. Brighton, UK: Institute of Development Studies. https://opendocs.ids.ac.uk/opendocs/handle/20.500.12413/13013
Modra, H., Palikova, M., Hyrsl, P., Bartonkova, J., Papezikova, I., Svobodova, Z., Blahova, J. and Mares, J., 2020. Effects of trichothecene mycotoxin T-2 toxin on haematological and immunological parameters of rainbow trout (Oncorhynchus mykiss). Mycotoxin Research, 36(3), 319-326.
Moldal, T., Bernhoft, A., Rosenlund, G., Kaldhusdal, M. and Koppang, E.O., 2018. Dietary deoxynivalenol (DON) may impair the epithelial barrier and modulate the cytokine signaling in the intestine of Atlantic salmon (Salmo salar). Toxins, 10(9), 376.
Nomura, H., Ogiso, M., Yamashita, M., Takaku, H., Kimura, A., Chikasou, M., Nakamura, Y., Fujii, S., Watai, M. and Yamada, H., 2011. Uptake by dietary exposure and elimination of aflatoxins in muscle and liver of rainbow trout (Oncorhynchus mykiss). Journal of Agricultural and Food Chemistry, 59(9), 5150-5158.
Ostrowski-Meissner HT, LeaMaster BR, Duerr EO, Walsh WA (1995) Sensitivity of the Pacific white shrimp, Penaeus vannamei, to aflatoxin B1. Aquaculture, 131: 155-164.
Pestka, J.J., 2007. Deoxynivalenol: Toxicity, mechanisms and animal health risks. Animal feed science and technology, 137(3-4), 283-298.
Pietsch, C., 2017. Zearalenone (ZEN) and its influence on regulation of gene expression in carp (Cyprinus carpio L.) liver tissue. Toxins, 9(9), 283.
Pietsch, C., Junge, R. and Burkhardt-Holm, P., 2015. Immunomodulation by zearalenone in carp (Cyprinus carpio L.). BioMed Research International, 2015, 420702.
Pinotti, L., Ottoboni, M., Giromini, C., Dell’Orto, V. and Cheli, F., 2016. Mycotoxin contamination in the EU feed supply chain: A focus on cereal byproducts. Toxins, 8(2), 45.
Placinta, C.M., D’Mello, J.F. and Macdonald, A.M.C., 1999. A review of worldwide contamination of cereal grains and animal feed with Fusarium mycotoxins. Animal Feed Science and Technology, 78(1-2), 21-37.
Poston, H.A., Coffin, J.L. and Combs, G.F., 1982. Biological effects of dietary T-2 toxin on rainbow trout, Salmo gairdneri. Aquatic Toxicology, 2(2), 79-88.
Sahoo, P.K., Mukherjee, S.C., Nayak, S.K. and Dey, S., 2001. Acute and subchronic toxicity of aflatoxin B1 to rohu, Labeo rohita (Hamilton). Indian Journal of Experimental Biology, 39(5), 453-458.
Santacroce, M. P., M. C. Conversano, E. Casalino, O. Lai, C. Zizzadoro, G. Centoducati, and G. Crescenzo. 2008. Aflatoxins in aquatic species: metabolism, toxicity and perspectives. Reviews in Fish Biology and Fisheries, 18:99-130.
Schwartz, P., Thorpe, K.L., Bucheli, T.D., Wettstein, F.E. and Burkhardt-Holm, P., 2010. Short-term exposure to the environmentally relevant estrogenic mycotoxin zearalenone impairs reproduction in fish. Science of the Total Environment, 409(2), 326-333.
Selim, K.M., El-hofy, H. and Khalil, R.H., 2014. The efficacy of three mycotoxin adsorbents to alleviate aflatoxin B1-induced toxicity in Oreochromis niloticus. Aquaculture International, 22(2), 523-540.
Shahafve, S., Banaee, M., Haghi, B.N. and Mohiseni, M., 2017. Histopathological study of common carp (Cyprinus carpio) fed aflatoxin-contaminated diets. International Journal of Aquatic Biology, 5(2), 63-70.
Subasinghe, R., Soto, D. and Jia, J. 2009. Global aquaculture and its role in sustainable development. Reviews in Aquaculture, 1(1), 2-9.
Tamm, C. and Breitenstein, W., 1984. The biosynthesis of mycotoxins. In: Mycotoxins: A Study in Secondary Metabolism, Ed.: Steyn, P.S., pp: 69-91. Academic Press, New York, NY.
Tilman, D. and Clark, M. 2014. Global diets link environmental sustainability and human health. Nature, 515, 518-522.
Tuan, N.A., Manning, B.B., Lovell, R.T. and Rottinghaus, G.E., 2003. Responses of Nile tilapia (Oreochromis niloticus) fed diets containing different concentrations of moniliformin or fumonisin B1. Aquaculture, 217(1-4), 515-528.
Varga, J., Frisvad, J. and Samson, R., 2009. A reappraisal of fungi producing aflatoxins. World Mycotoxin Journal, 2(3), 263-277.
Wang, E., Ross, P.F., Wilson, T.M., Riley, R.T. and Merrill Jr, A.H., 1992. Increases in serum sphingosine and sphinganine and decreases in complex sphingolipids in ponies given feed containing fumonisins, mycotoxins produced by Fusarium moniliforme. The Journal of Nutrition, 122(8), 1706-1716.
Wang, Y., Wang, B., Liu, M., Jiang, K., Wang, M. and Wang, L., 2018. Aflatoxin B1 (AFB1) induced dysregulation of intestinal microbiota and damage of antioxidant system in Pacific white shrimp (Litopenaeus vannamei). Aquaculture, 495, 940-947.
Woźny, M., Dobosz, S., Obremski, K., Hliwa, P., Gomułka, P., Łakomiak, A., Różyński, R., Zalewski, T. and Brzuzan, P., 2015. Feed-borne exposure to zearalenone leads to advanced ovarian development and limited histopathological changes in the liver of premarket size rainbow trout. Aquaculture, 448, 71-81.
Wu, T.S., Cheng, Y.C., Chen, P.J., Huang, Y.T., Yu, F.Y. and Liu, B.H., 2019. Exposure to aflatoxin B1 interferes with locomotion and neural development in zebrafish embryos and larvae. Chemosphere, 217, 905-913.
Yildirim, M., Manning, B.B., Lovell, R.T., Grizzle, J.M. and Rottinghaus, G.E., 2000. Toxicity of moniliformin and fumonisin B1 fed singly and in combination in diets for young channel catfish Ictalurus punctatus. Journal of the World Aquaculture Society, 31(4), 599-608.
Zain, M.E., 2011. Impact of mycotoxins on humans and animals. Journal of Saudi Chemical Society, 15(2), 129-144.
Zhang, Y., Rossi, W., Yamamoto, F.Y., Velasquez, A.M., Wang, A. and Gatlin, D.M., 2022. Effects of Dietary Aflatoxin B1 on Hybrid Striped Bass (Morone chrysops× M. saxatilis) and Assessment of Supplemental Arginine as a Potential Aflatoxicosis Alleviator. Aquaculture Nutrition, 5161222.