Prof. Naresh Magan and Dr. Ángel Medina
Applied Mycology Group, Environment and Agrifood Theme, Cranfield University, Cranfield, MK43 0AL, U.K.
Email: [email protected]
However, when we examine the ingredients which are used, especially in Europe, a significant number of these are rejected at the borders because of mycotoxins, especially those originating from sub-tropical and tropical regions (RASFF, 2019).
We will address the use of such preservatives and their potential drawbacks, especially when using sub-optimal concentrations which may exacerbate mycotoxin contamination.
There has also been a lot of interest in finding alternative food/feed grade preservatives based on antioxidants and plant extracts, especially essential oils. However, many of these are not classed as GRAS compounds because the necessary toxicology has not been completed and in addition the cost-benefit analyses needs to be included to evaluate their potential for commercial use. These aspects will also be addressed.
ALIPHATIC ACIDS AND THEIR SALTS FOR CONTROLLING SPOILAGE AND MYCOTOXIGENIC MOULDS
Animal feed consists of mixtures of ingredients including cereals, cereal by-products, cotton seed cake, groundnut cake, soya extracts, palm pulp, pulses and other additives depending on whether the product is destined for cattle, swine, or poultry.
The production of pelleted feed results in a relatively dry feed product that can be stored effectively in the short and medium term, provided they do not absorb moisture during the storage phase, due to poor management post-harvest.
This can occur because of ineffective partitioning of different types of commodities during transport or storage, since feed ingredients can re-absorb moisture. This is especially so if some of the ingredients are hygroscopic, which can allow the initiation of growth by spoilage and indeed mycotoxigenic moulds resulting in contamination with mycotoxins (Perreira et al., 2019).
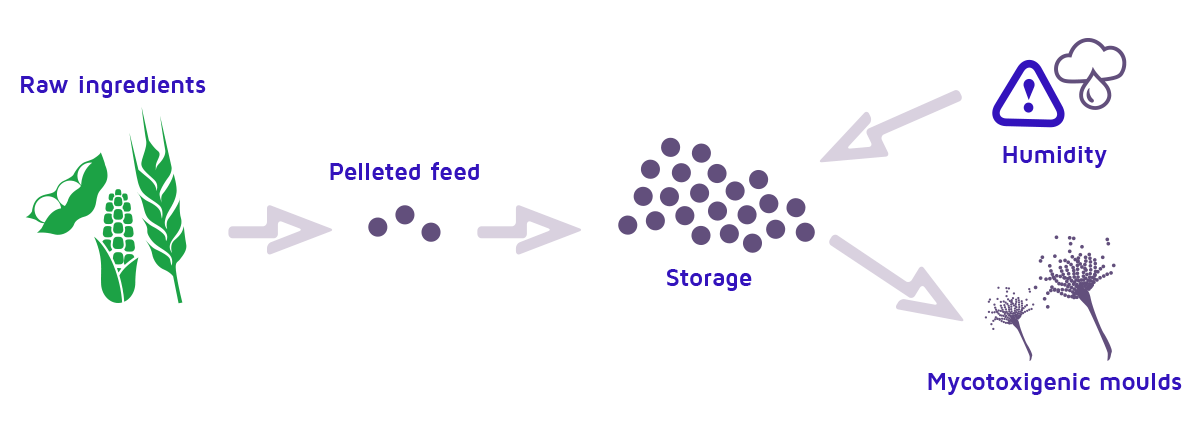
Economically, spoilage moulds and mycotoxin contamination are very important.
In a 10-year study in the USA, it was shown that animal feed ingredients and forage samples contained significant amounts of mycotoxins. For example:
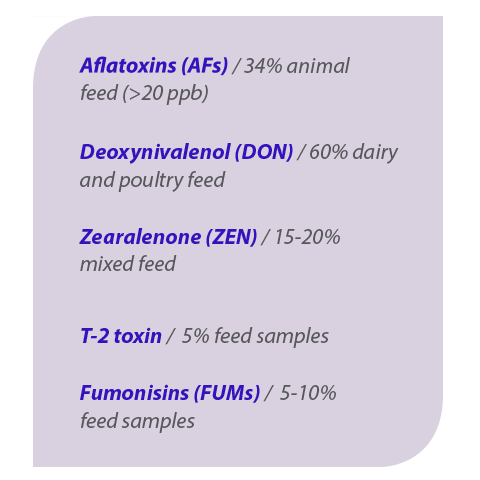
Forages including concentrates, maize grain, soybean meal, cottonseed cake, corn silage, grass hay, small grain and grass silage have been surveyed for the presence of mycotoxins.
These have all shown a significant increase over recent years in the percentage of samples of feed which are contaminated with different mycotoxins including those by Biomin, Alltech, Randox and Patent Co, entre otras (Biomin survey, 2019; Alltech survey, 2019; Randox survey, 2019, Patent Co 2019).
Non-extruded feedstuffs, including hay and mixed feeds, are often treated with anti-mould preservatives, usually based on individual or mixtures of aliphatic acids or their salts. This can reduce the potential for initiation of mould spoilage and mycotoxin contamination.
Most of the preservative mixtures used commercially are, however, fungistatic not fungicidal, and thus are not lethal to contaminating mould spores.
Under-treated or non-treated pockets of feed, even very small ones, of a few grams, may provide initial foci for the initiation of germination of spoilage/mycotoxigenic mould spores if the feed moisture content is conducive to growth (Lord et al., 1981, 1983; Lacey et al., 1982).
It is thus critical that, when preservatives are added, effective mixing of the preservative with the feed is achieved to ensure coverage of the feed with the right concentration to minimize opportunities for spoilage moulds/ mycotoxigenic mould initiation and toxin contamination from occurring during short- and medium-term storage.
Indeed, elegant studies by Lord et al. (1981) showed the importance of effective mixing of aliphatic acids or their salts with the feed to prevent undertreated or untreated pockets of the feed.
They suggested that mould spoilage may be initiated as well as contamination with aflatoxins (AFs; class 1a carcinogens), other mycotoxins such as ochratoxin A (OTA) or type B trichothecenes, including DON, ZEN, and FUMs.
Studies of different poultry mix feeds used as starter and finisher blends were shown to have different mycobiota diversity depending on the season and the way the feed raw ingredients are processed.
Often the predominant spoilage mould species were from the Aspergillus, Penicillium and Fusarium, responsible for mycotoxin contamination, especially in warmer regions of the world, e.g., South-East Asia (Alam et al., 2010 a,b).
In Asia, poultry feed is produced by commercial feed mills as well as by local cooperatives. The feed mill owners purchase these ingredients in bulk in the production season and store them for processing into feed throughout the year.
However, often in tropical and sub-tropical climatic regions, harvested raw commodities from the fields often have a relatively high moisture content, which makes them unsafe for even short-term storage without mould spoilage. Thus, without effective drying to <0.70 water activity, then intermediate moisture conditions will allow the development of dry loving (xerophilic) moulds mentioned previously, many of which are mycotoxigenic (Ilangantileke, 1987; Sanchis, 2000; Figure 1).
A number of commercial companies sell preservative formulations based on aliphatic acids including formic, propionic, or sorbic acids or mixtures thereof.
Sometimes their salts are preferred because they have a reduced volatility and are more user friendly.
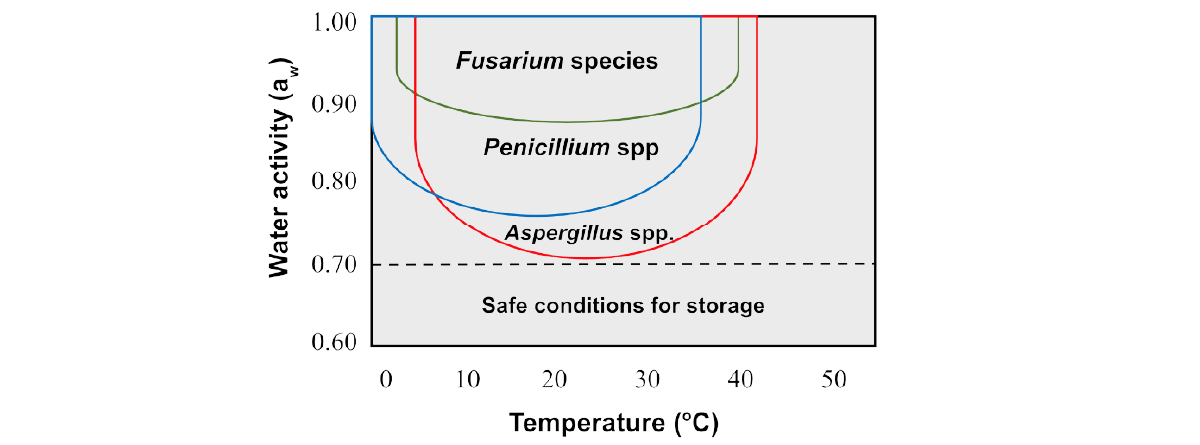
Figure 1. Diagrammatic profiles of growth/no growth limits for the three key mycotoxigenic genera in relation to the two key interaction abiotic factors.