S. Divyashree, B. Shruthi and M.Y. Sreenivasa*
Applied Mycology Lab, Department of Studies in Microbiology, University of Mysore, Mysuru-570006, Karnataka, India.
*Corresponding author e-mail: [email protected]; [email protected]
Mycotoxins are toxic metabolites produced by fungi, which can cause both acute and chronic diseases in humans and animals.
They are lipophilic molecules primarily absorbed in the gut and later distributed to fat and other soft tissues, leading to kidney and liver damage.
The International Agency for Research on Cancer (IARC) has classified some mycotoxins, such as aflatoxins, fumonisins, and ochratoxins, as Group 2B carcinogens.
Mycotoxins also contaminate crops such as cereals, nuts, seeds, vegetables, and fruits, causing significant losses in the agricultural sector.
The Food and Agriculture Organization (FAO) estimates that 25 % of global agricultural crops are affected by mycotoxins (Eskola et al., 2020).
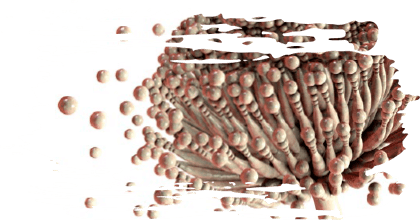
Over time, mycotoxins have received considerable attention, especially over the last three decades and, as a result, the field of mycotoxicology is gaining more importance due to its toxicological effects (Sreenivasa et al., 2008 y 2011).
Mycotoxicosis is the illness caused by the consumption of mycotoxins by humans and animals through toxin-contaminated food and feed, which can lead to immune suppression and, even, cancer.
Additionally, mycotoxin intoxication in livestock results in significant economic losses in the meat industry (Nagaraja, 2016).
To decontaminate mycotoxins, various detoxification methods are employed, including physical, chemical, and biological strategies (Deepa & Sreenivasa, 2019).
- ⇰ Physical methods, such as heat treatment and adsorption, are quick but may affect the quality and nutritional value of food.
- ⇰ Chemical techniques, such as ammonification and ozonation, are effective but can pose food safety risks.
To address these challenges, biological detoxification has emerged as a viable alternative, utilizing microorganisms and their products. Additionally, enzymatic degradation presents a promising strategy for selective detoxification, offering an eco-friendly, reusable, and effective solution.
This method ensures safety for human and animal health while supporting sustainable agricultural practices (Deepa et al., 2017).
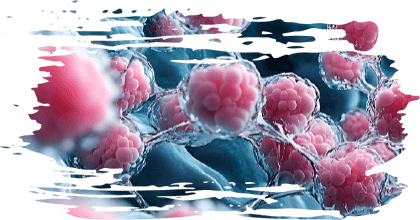
Mycotoxins and their properties
Mycotoxins are classified into various groups based on:
Chemical structure
Biological activity
Fungi responsible for their production
An outline of their classification and toxicological effects is listed in Table 1.
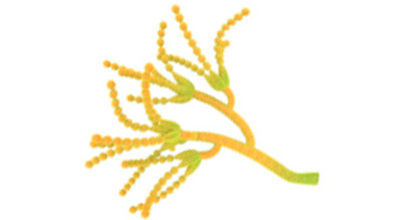
Table 1. Some of the major mycotoxins produced by fungi and their toxic effects.
Enzymatic degradation mechanisms
Currently, biological degradation strategies have gained more popularity over physical and chemical methods.
The biological degradation of mycotoxins involves active metabolites secreted by microorganisms which alter the structure of mycotoxins, converting them into less toxic or non-toxic products (Figure 1).
Figure 1. Conversion of mycotoxins into less toxic compounds by enzymes.
Enzymatic degradation of mycotoxins offers various advantages over microbial degradation, including high specificity, efficiency, performance, and ease of implementation (Ji et al., 2016).
These mycotoxin-degrading enzymes provide a major advantage over other detoxification methods by ensuring the safe and effective removal of these harmful substances.
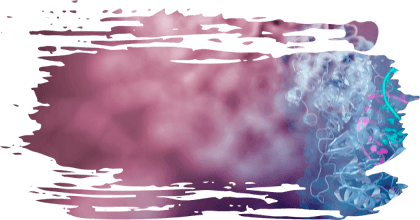
The degradation of mycotoxins can occur either extracellularly or intracellularly (Figure 2).
- ⇰ Extracellular degradation involves the release of enzymes or metabolites by microorganisms that break down mycotoxins outside the cell.
- ⇰ Intracellular degradation occurs when microorganisms absorb mycotoxins, and enzymes inside the cell modify or degrade these compounds.
Both processes contribute to mycotoxin detoxification, with extracellular degradation often being faster and more efficient in some cases.
Figure 2. Intracellular and extracellular enzymatic degradation of mycotoxins.
A list of enzymes that degrade mycotoxins is provided in Table 2.
Table 2. List of enzymes involved in mycotoxin degradation.
Recent research has focused on identifying and developing effective enzymatic strategies for mycotoxin degradation, aiming to mitigate their impact on food and feed safety.
Various enzymes from microorganisms and fungi have shown promising results in breaking down aflatoxins into less toxic compounds, offering environmentally friendly and efficient detoxification methods.
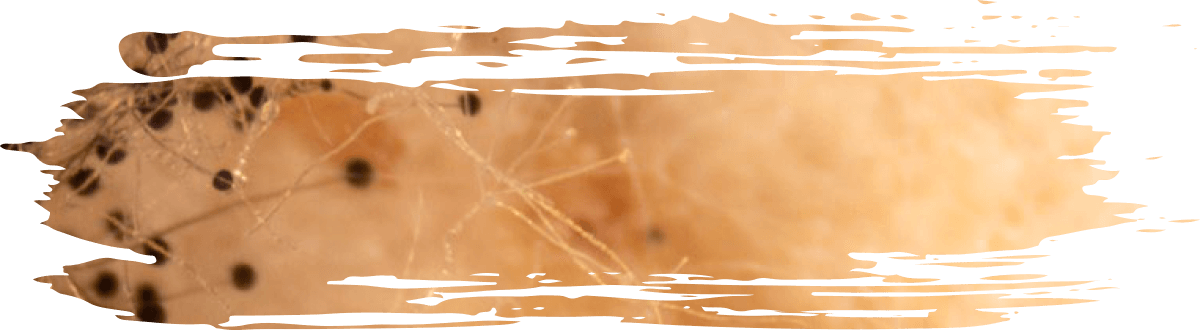
Aflatoxin degradation
Several notable studies have demonstrated the potential of enzymatic degradation, highlighting different sources of aflatoxin-degrading enzymes and their mechanisms of action.
A strategy was developed to express an aflatoxin-degrading enzyme from the edible honey mushroom Armillariella tabescens in developing maize kernels, effectively controlling aflatoxin contamination under harvest conditions ( (Schmidt et al., 2021).
Ligninolytic enzymes from spent mushroom substrate (SMS), a by-product of edible mushroom cultivation, demonstrated over 90 % degradation of aflatoxin B1 (AFB1) after seven days, with optimal degradation occurring at pH 8 (Brana et al., 2019).
The extracellular enzyme MADE (Mycobacteria Aflatoxin Degradation Enzyme), isolated from Myxococcus fulvus ANSMO68, has shown high efficacy in degrading aflatoxins B1, G1, and M1, achieving 72 % degradation of AFB1, 68 % of AFG1, and 64 % of AFM1 after 48 hours (Zhao et al., 2010).
The dye-decoloring peroxidase (Dyp) from Bacillus subtilis SCK6, expressed in E. coli, efficiently degraded aflatoxin B1 in the presence of Mn2+ (Qin et al., 2021).
Ochratoxin degradation
Enzymatic strategies have also been explored for the breakdown of ochratoxins, another group of mycotoxins of major concern in food and feed safety.
Several studies have investigated the potential of fungal and plant-derived enzymes to degrade ochratoxin A (OTA) efficiently, highlighting their application in sustainable detoxification approaches.
A study on exoenzymes from Trichoderma afroharzianum strain T22, or its peroxidase supplementation, demonstrated significant degradation of both aflatoxin B1 and ochratoxin A, providing a sustainable method for detoxifying mycotoxin-contaminated food and feed (Dini et al., 2022).
The commercial peroxidase enzyme (POD) from Armoracia rusticana was evaluated for its ability to degrade both ochratoxin A (OTA) and zearalenone (ZEN), achieving 27 % and 48 % degradation of OTA and ZEN, respectively, in beer after 6 hours (Garcia et al., 2020).
Zearalenone degradation
In addition to aflatoxins and ochratoxins, zearalenone (ZEN) is another mycotoxin of significant concern, particularly due to its estrogenic effects in livestock.
Research has focused on enzymatic approaches to degrade ZEN efficiently, with promising results from both recombinant and plant-derived enzymes.
A recombinant fusion enzyme, combining zearalenone hydrolase (ZHD) and carboxypeptidase (CP), was developed to achieve 100 % degradation of ZEN within 2 hours at pH 7 and 35 °C. In contrast, CP alone degraded only 60 % of ZEN in 28 hours. The combination of ZHD-CP and CP also achieved 100 % degradation of OTA in just 30 minutes (Azam et al., 2019).
Armoracia rusticana exhibited substantial ZEN degradation, reaching 64.9 % degradation in a model solution after 6 hours (Garcia et al., 2020).
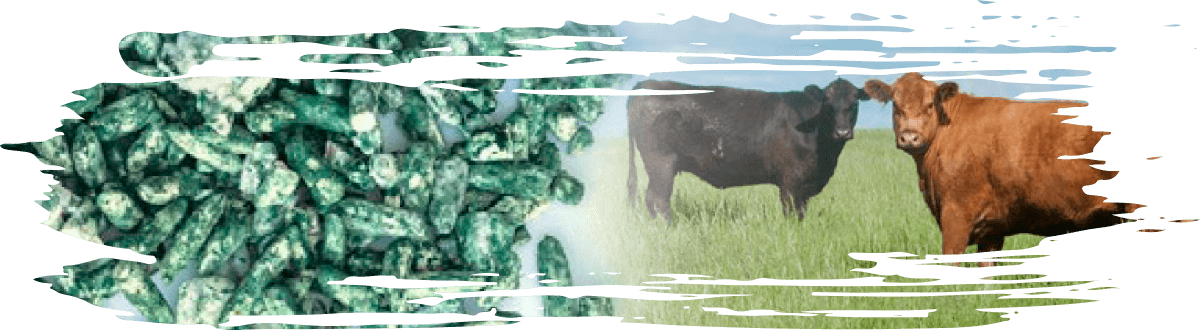
Fumonisin degradation
Fumonisins are another group of mycotoxins of concern, particularly due to their impact on animal health and their association with diseases such as leukoencephalomalacia in horses and pulmonary edema in pigs.
Recent research has explored bacterial strains and enzymatic mechanisms capable of degrading fumonisins effectively.
Recombinant carboxylesterase was used to catalyse the deesterification of fumonisin B1 to hydrolysed fumonisin B1. When supplemented at 60 U/kg, this enzyme significantly reduced fumonisin B1 levels in turkeys and pigs (Masching et al., 2016). This suggests that recombinant enzymes have potential for detoxifying fumonisin-contaminated feed and food.
Carboxylesterases and aminotransferases have been used to synergistically degrade fumonisins.
Deoxynivalenol degradation
Deoxynivalenol (DON), also known as vomitoxin, is a mycotoxin commonly found in cereals, posing a significant risk to animal health and feed safety.
Recent studies have focused on enzymatic and microbial strategies to degrade or detoxify DON, converting it into less toxic compounds.
A novel bacterial strain, Pelagibacterium halotolerans ANSP101, isolated from seawater, successfully transformed DON into the less toxic 3-keto-deoxynivalenol by oxidizing the C3 hydroxyl group. This DON-degrading activity was attributed to intracellular proteins, with optimal degradation occurring in cereals and feed (Zhang et al., 2020).
Dehydrogenases, reductases, glyoxalases, and acyltransferases have been effectively used to detoxify or convert DON into non-toxic intermediates.
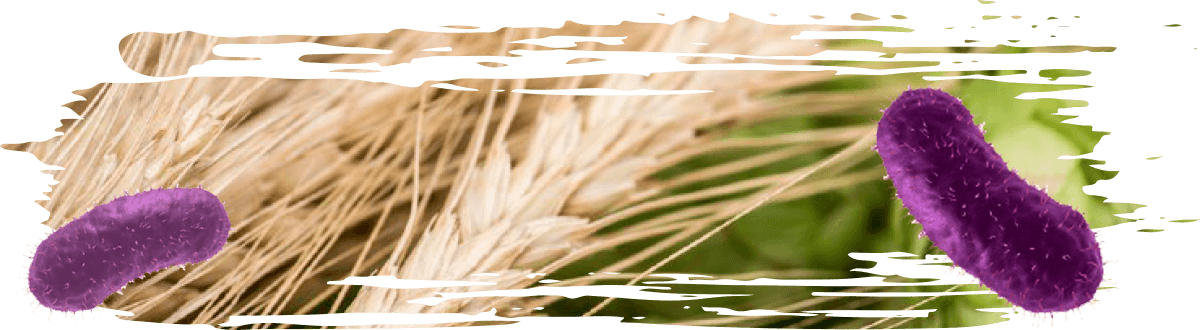
CONCLUSION
In conclusion, the widespread contamination of food and feed by mycotoxins has drawn global attention.
Various strategies have been developed to mitigate this issue, including physical and chemical methods, with biological detoxification emerging as a safe and effective alternative.
The use of microorganisms for mycotoxin detoxification represents a promising approach for the food and feed industries, particularly through enzymatic degradation.
This method is valued for its specificity, effectiveness, environmental friendliness, and safety.
Specific enzymes play a crucial role in converting toxic mycotoxins into less harmful or non-toxic compounds.
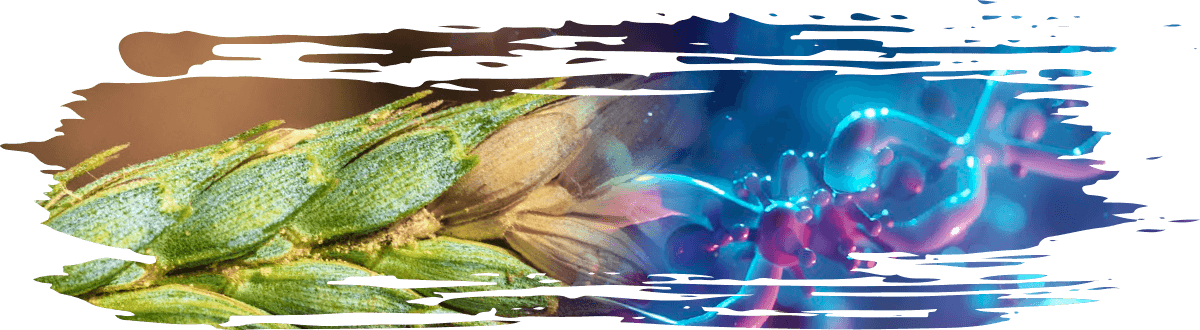
REFERENCES
1. Accinelli, C., Abbas, H.K., Vicari, A. and Shier, W.T., 2014. Aflatoxin contamination of corn under different agro-environmental conditions and biocontrol applications. Crop Protection, 63, pp.9-14.
2. Abrunhosa, L., Venâncio, A. and Teixeira, J.A., 2011. Optimization of process parameters for the production of an OTA-hydrolyzing enzyme from Aspergillus niger under solid-state fermentation. Journal of bioscience and bioengineering, 112(4), pp.351-355. https://doi.org/10.1016/j.jbiosc.2011.06.016
3. Azam, M.S., Yu, D., Liu, N. and Wu, A., 2019. Degrading ochratoxin A and zearalenone mycotoxins using a multifunctional recombinant enzyme. Toxins, 11(5), p.301. https://doi.org/10.3390/toxins11050301
4. Adithi G, Rakesh Somashekaraiah, S. Divyashree, B. Shruthi, M Y Sreenivasa 2022. Assessment of probiotic and antifungal activity of Lactiplantibacillus plantarum MYSAGT3 isolated from locally available herbal juice against mycotoxigenic Aspergillus species. Food Bioscience. 102118.
5. Boesch-Saadatmandi, C., Loboda, A., Józkowicz, A., Huebbe, P., Blank, R., Wolffram, S., Dulak, J. and Rimbach, G., 2008. Effect of ochratoxin A on redox-regulated transcription factors, antioxidant enzymes and glutathione-S-transferase in cultured kidney tubulus cells. Food and Chemical Toxicology, 46(8), pp.2665-2671. https://doi.org/10.1016/j.fct.2008.04.023
6. Branà, M.T., Sergio, L., Haidukowski, M., Logrieco, A.F. and Altomare, C., 2020. Degradation of aflatoxin B1 by a sustainable enzymatic extract from spent mushroom substrate of Pleurotus eryngii. Toxins, 12(1), p.49. https://doi.org/10.3390/toxins12010049
7. Deepa N and M Y Sreenivasa. 2017. Fumonisins: A Review on its Global Occurrence, Epidemiology, Toxicity and Detection. Journal of Veterinary Medicine and Research 4(6): 1093.
8. Deepa, N., Nagaraja, H. and Sreenivasa, M.Y., 2016. Prevalence of fumonisin producing Fusarium verticillioides associated with cereals grown in Karnataka (India). Food Science and Human Wellness, 5(3), pp.156-162. https://doi.org/10.1016/j.fshw.2016.07.001
9. Deepa N and M.Y. Sreenivasa. 2019. Biocontrol Strategies for Effective Management of Phytopathogenic Fungi Associated With Cereals, In: Singh JS., Singh DP. (eds) New and Future Developments in Microbial Biotechnology and Bioengineering, Elsevier, pp, 177-189, https://doi.org/10.1016/B978-0-444-64191-5.00013-4
10. de Oliveira Garcia, S., Sibaja, K.V.M., Nogueira, W.V., Feltrin, A.C.P., Pinheiro, D.F.A., Cerqueira, M.B.R., Furlong, E.B. and Garda-Buffon, J., 2020. Peroxidase as a simultaneous degradation agent of ochratoxin A and zearalenone applied to model solution and beer. Food Research International, 131, p.109039.
11. Dini, I., Alborino, V., Lanzuise, S., Lombardi, N., Marra, R., Balestrieri, A., Ritieni, A., Woo, S.L. and Vinale, F., 2022. Trichoderma enzymes for degradation of aflatoxin B1 and ochratoxin A. Molecules, 27(12), p.3959.
12. Eskola, M., Kos, G., Elliott, C.T., Hajšlová, J., Mayar, S. and Krska, R., 2020. Worldwide contamination of food-crops with mycotoxins: Validity of the widely cited ‘FAO estimate’of 25%. Critical reviews in food science and nutrition, 60(16), pp.2773-2789. https://doi.org/10.1080/10408398.2019.1658570
13. Fang, J., Sheng, L., Ye, Y., Ji, J., Sun, J., Zhang, Y. and Sun, X., 2023. Recent advances in biosynthesis of mycotoxin-degrading enzymes and their applications in food and feed. Critical Reviews in Food Science and Nutrition, pp.1-17.
14. Guo, W., Zhao, M., Chen, Q., Huang, L., Mao, Y., Xia, N., Teng, J. and Wei, B., 2019. Citrinin produced using strains of Penicillium citrinum from Liupao tea. Food Bioscience, 28, pp.183-191.
15. Heinl, S., Hartinger, D., Thamhesl, M., Vekiru, E., Krska, R., Schatzmayr, G., Moll, W.D. and Grabherr, R., 2010. Degradation of fumonisin B1 by the consecutive action of two bacterial enzymes. Journal of biotechnology, 145(2), pp.120-129.
16. Igawa, T., Takahashi-Ando, N., Ochiai, N., Ohsato, S., Shimizu, T., Kudo, T., Yamaguchi, I. and Kimura, M., 2007. Reduced contamination by the Fusarium mycotoxin zearalenone in maize kernels through genetic modification with a detoxification gene. Applied and Environmental Microbiology, 73(5), pp.1622-1629.
17. Ito, M., Sato, I., Ishizaka, M., Yoshida, S.I., Koitabashi, M., Yoshida, S. and Tsushima, S., 2013. Bacterial cytochrome P450 system catabolizing the Fusarium toxin deoxynivalenol. Applied and environmental microbiology, 79(5), pp.1619-1628.
18. Janik, E., Niemcewicz, M., Podogrocki, M., Ceremuga, M., Stela, M. and Bijak, M., 2021. T-2 toxin—The most toxic trichothecene mycotoxin: Metabolism, toxicity, and decontamination strategies. Molecules, 26(22), p.6868.
19. Ji, C., Fan, Y. and Zhao, L., 2016. Review on biological degradation of mycotoxins. Animal nutrition, 2(3), pp.127-133.
20. Liu, M., Zhang, X., Luan, H., Zhang, Y., Xu, W., Feng, W. and Song, P., 2024. Bioenzymatic detoxification of mycotoxins. Frontiers in microbiology, 15, p.1434987. https://doi.org/10.3389/fmicb.2024.1434987
21. Maiorano, A., Blandino, M., Reyneri, A. and Vanara, F., 2008. Effects of maize residues on the Fusarium spp. infection and deoxynivalenol (DON) contamination of wheat grain. Crop Protection, 27(2), pp.182-188. 007. doi:10.1016/j.cropro.2007.05.004
22. Miedaner, T. and Geiger, H.H., 2015. Biology, genetics, and management of ergot (Claviceps spp.) in rye, sorghum, and pearl millet. Toxins, 7(3), pp.659-678.
23. Nagaraja H., Chennappa G., Poorna Chandra Rao K., Mahadevprasad G., and M Y Sreenivasa. 2016. Diversity of toxic and phytopathogenic Fusarium species occurring on cereals grown in Karnataka state, India. 3Biotech. 6:57
24. Nagaraja H., Chennappa G., Rakesh S, Naik MK, Amaresh YS and M. Y. Sreenivasa. 2016. Antifusarial activity of Azotobacter nigricans against trichothecene-producing Fusarium species associated with cereals. Food Science and Biotechnology. 25(4): 1197-1204. DOI 10.1007/s10068-016-0.
25. Ostry, V., 2008. Alternaria mycotoxins: an overview of chemical characterization, producers, toxicity, analysis and occurrence in foodstuffs. World Mycotoxin Journal, 1(2), pp.175-188.
26. Pereyra, M.G., Martínez, M.P. and Cavaglieri, L.R., 2019. Presence of aiiA homologue genes encoding for N-Acyl homoserine lactone-degrading enzyme in aflatoxin B1 decontaminating Bacillus strains with potential use as feed additives. Food and Chemical Toxicology, 124, pp.316-323. https://doi.org/10.1016/j.fct.2018.12.016
27. Qin, X., Su, X., Tu, T., Zhang, J., Wang, X., Wang, Y., Wang, Y., Bai, Y., Yao, B., Luo, H. and Huang, H., 2021. Enzymatic degradation of multiple major mycotoxins by dye decolorizing peroxidase from Bacillus subtilis. Toxins, 13(6), p.429.
28. Saleh, I. and Goktepe, I., 2019. The characteristics, occurrence, and toxicological effects of patulin. Food and chemical toxicology, 129, pp.301-311.
29. Scharf, D.H., Heinekamp, T., Remme, N., Hortschansky, P., Brakhage, A.A. and Hertweck, C., 2012. Biosynthesis and function of gliotoxin in Aspergillus fumigatus. Applied microbiology and biotechnology, 93, pp.467-472.
30. Schmidt, M.A., Mao, Y., Opoku, J. and Mehl, H.L., 2021. Enzymatic degradation is an effective means to reduce aflatoxin contamination in maize. BMC biotechnology, 21, pp.1-10. https://doi.org/10.1186/s12896-021-00730-6
31. Sreenivasa M.Y., Regina Sharmila Dass, A. P. Charith Raj and G. R. Janardhana. 2011. Mycological evaluation of Maize grains produced in Karnataka (India) for the post harvest fungal contamination. World Applied Sciences Journal, 13(4), 688 – 692.
32. Sreenivasa M Y, Regina Sharmila Dass, A. P. Charith Raj and G. R. Janardhana. 2008. PCR-based detection of genus Fusarium and Fumonisin-producing isolates from freshly harvested Sorghum grains grown in Karnataka, India. Journal of Food Safety, 28: 236-237.
33. Silva, A.C. and Venâncio, A., 2021. Application of laccases for mycotoxin decontamination. World Mycotoxin Journal, 14(1), pp.61-74.
34. Welsch, T. and Humpf, H.U., 2012. HT-2 toxin 4-glucuronide as new T-2 toxin metabolite: enzymatic synthesis, analysis, and species specific formation of T-2 and HT-2 toxin glucuronides by rat, mouse, pig, and human liver microsomes. Journal of Agricultural and Food Chemistry, 60(40), pp.10170-10178. doi.org/10.1021/jf302571y l
35. Zhang, J., Fang, Y., Fu, Y., Jalukar, S., Ma, J., Liu, Y., Guo, Y., Ma, Q., Ji, C. and Zhao, L., 2023. Yeast polysaccharide mitigated oxidative injury in broilers induced by mixed mycotoxins via regulating intestinal mucosal oxidative stress and hepatic metabolic enzymes. Poultry Science, 102(9), p.102862. https://doi.org/10.1016/j.psj.2023.102862
36. Zhang, J., Qin, X., Guo, Y., Zhang, Q., Ma, Q., Ji, C. and Zhao, L., 2020. Enzymatic degradation of deoxynivalenol by a novel bacterium, Pelagibacterium halotolerans ANSP101. Food and Chemical Toxicology, 140, p.111276. https://doi.org/10.1016/j. fct.2020.111276 Get rights and content
37. Zhao, L.H., Guan, S., Gao, X., Ma, Q.G., Lei, Y.P., Bai, X.M. and Ji, C., 2011. Preparation, purification and characteristics of an aflatoxin degradation enzyme from Myxococcus fulvus ANSM068. Journal of Applied Microbiology, 110(1), pp.147-155. https://doi. org/10.1111/j.1365-2672.2010.04867.x