Al-Zahraa Mamdouh1 and Eman Zahran2*
1Fish Diseases Department, National Institute of Oceanography and Fisheries (NIOF), Egypt
2Department of Internal Medicine, Infectious and Fish Diseases, Faculty of Veterinary Medicine, Mansoura University, Mansoura, Egypt
*Corresponding author: [email protected]
Mycotoxins are toxic substances produced naturally by filamentous fungi as secondary metabolites that can be found in food for humans and feed for livestock.
Cereals, nuts, corn, and rice can be contaminated in the field during harvest or storage, making them a common source of contamination.
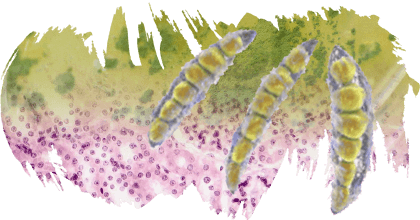

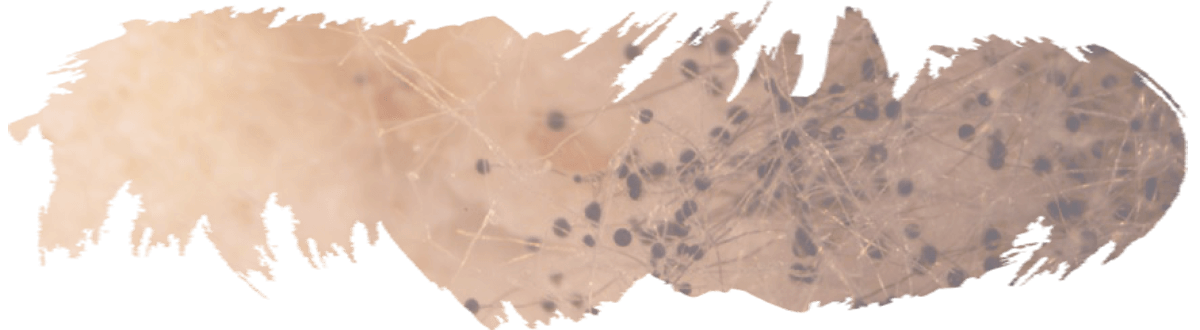
The threat of mycotoxins
Mycotoxins are produced naturally as secondary metabolites with no known metabolic function by filamentous fungi (Edite Bezerra da Rocha et al., 2014).
⇰ They are considered toxic substances when present in human food or animal feed.
They can appear during pre-harvest, post-harvest, processing, storage, and feeding. Besides, they are commonly found in human and animal food derivatives (Sforza et al., 2006).
These toxins are produced by fungal species belonging mainly to:
- ⇰ Aspergillus
- ⇰ Fusarium
- ⇰ Alternaria
- ⇰ Penicillium
- ⇰ Myrothecium
- ⇰ Trichothecium
- ⇰ Verticimonosporium
(Zain, 2011)
Mycotoxins are one of the main classes of naturally occurring toxic substances contaminating food and feed worldwide (Hueza et al., 2014; Shetty et al., 2006; Taheur et al., 2017).
According to the Food and Agriculture Organization (FAO), about 25% of the food produced in the world is contaminated with at least one mycotoxin (Rogowska et al., 2019).
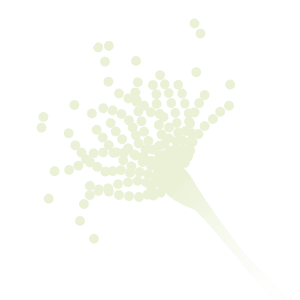
Contamination of animal and human food with mycotoxins occurs directly or indirectly.
⇰ Direct contamination occurs through the infection of the food by a toxigenic fungus with subsequent production of mycotoxins, whereas indirect contamination occurs through previous contamination of food ingredients by a toxigenic fungus, even though it has been eliminated during processing (Edite Bezerra da Rocha et al., 2014).
Factors enhancing mold growth and mycotoxin production include weather conditions, particularly humidity levels (above 70%) and temperature (20–30°C).
Additionally, the plant’s moisture content (above 15%), mechanical damage, damage to crops by insects, substrate composition, use of pesticides, plant variety, and spore load influence the production of mycotoxins (Streit et al., 2012).
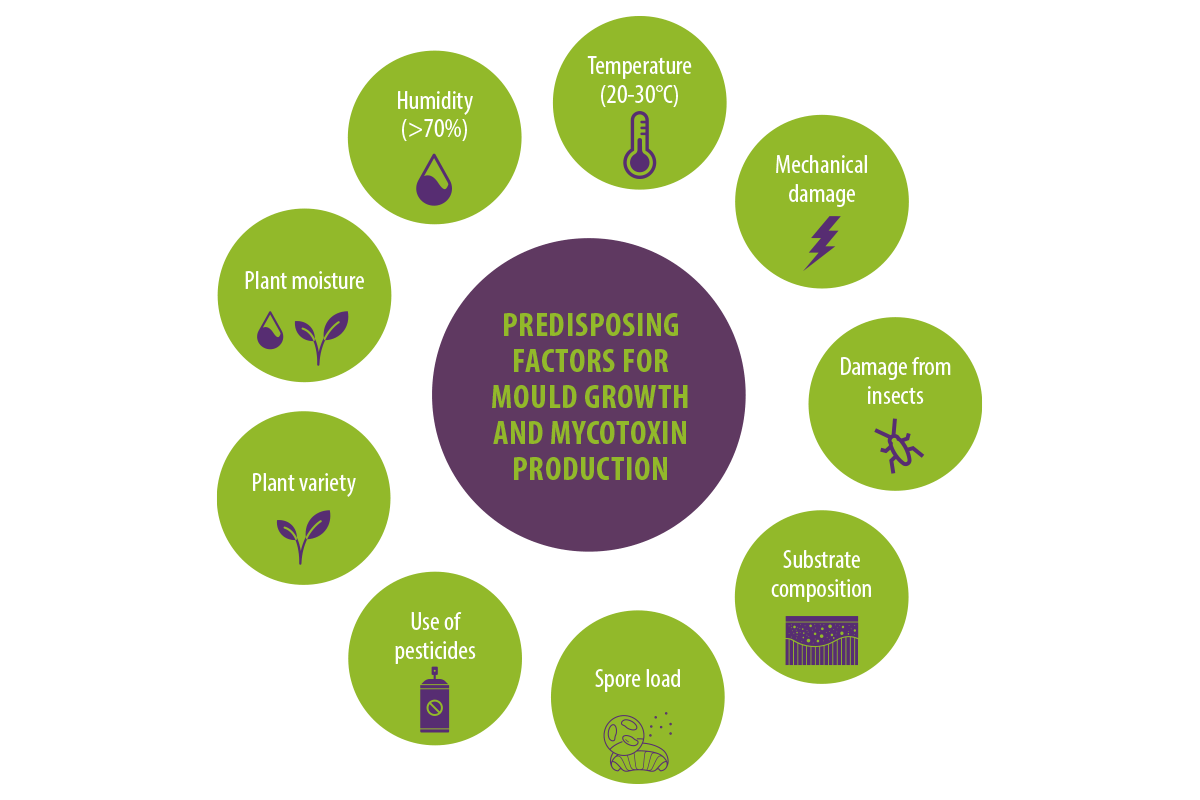
Mycotoxin-containing ingredients used in preparing food and feedstuffs have less nutritional value and represent a risk for animal and human health (Matejova et al., 2017). |
Major mycotoxins that pose a risk to human and animal health
Aflatoxins
Aflatoxins are difuranocoumarin derivatives that are produced in a variety of food, including maize, groundnuts, rice, sorghum (Kew, 2013), pistachio, groundnuts, tree nuts (almonds, walnut, hazelnut, brazil nuts), cottonseed, spices, dried fruits, cereals, soybean, cocoa, milk, milk products, and meat (Patriarca y Pinto, 2017; Vila-Donat et al., 2018).
⇰ They are produced mainly by Aspergillus flavus and A parasiticus and, to a lesser extent, A nomius, A bombycis, Aspergillus pseudotamari and Aspergillus ochraceoroseus (Varga et al., 2011).
Based on their blue or green fluorescence under ultraviolet light, the main known aflatoxins are B1, B2, G1 and G2.
AFB1 and AFB2 sare produced mainly by A. flavus, while others are produced by A. parasiticus (Dhanasekaran et al., 2011).
Other types of aflatoxins include P1, Q1, B2A and G2A, which are formed due to biotransformation or metabolism of aflatoxins in humans and animals (Doi et al., 2002).
Additionally, aflatoxin M1, a hydroxylated form of aflatoxin B1, is formed in animal tissues and fluids as a metabolite of AFB1 (Neal et al., 1998), whereas aflatoxin M2 is a metabolite of aflatoxin B2 formed in cattle milk (Dhanasekaran et al., 2011).
Several factors enhance the production of aflatoxins.
For example, tropical and sub-tropical regions characterized by high temperatures and humidity, along with moisture content of the plants, have favorable conditions for the growth of Aspergillus and the production of aflatoxins (Kew, 2013).
The most favorable temperature for their production is 25-32˚C, as well as moisture contents over 12% and less than 16%, and relative humidity of 85%.
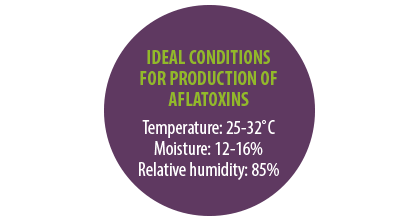
MECHANISMS OF TOXICITY AND EFFECTS
Aflatoxins are the most researched group of mycotoxins due to their carcinogenic and toxic effects in laboratory animals and livestock (Jha et al., 2013; Rotimi et al., 2017), as well as their acute and chronic hepatocarcinogenic and toxicological effects in human beings (Asim et al., 2011; Kew, 2013).
Aflatoxin toxicity arises mainly from the generation of intracellular reactive oxygen species (ROS), such as superoxide anion, hydroxyl radical, and hydrogen peroxide (H2O2), during its metabolism by cytochrome P450 in the liver.
⇰ These ROS target cellular DNA, RNA, proteins, and cell membranes, leading to the impairment of cell function, oxidative stress, DNA damage, cytolysis, and apoptosis (Asim et al., 2011; Yang et al., 2016). In addition, they can target the p53 tumor suppressor gene causing hepatocarcinoma (Kew, 2013).
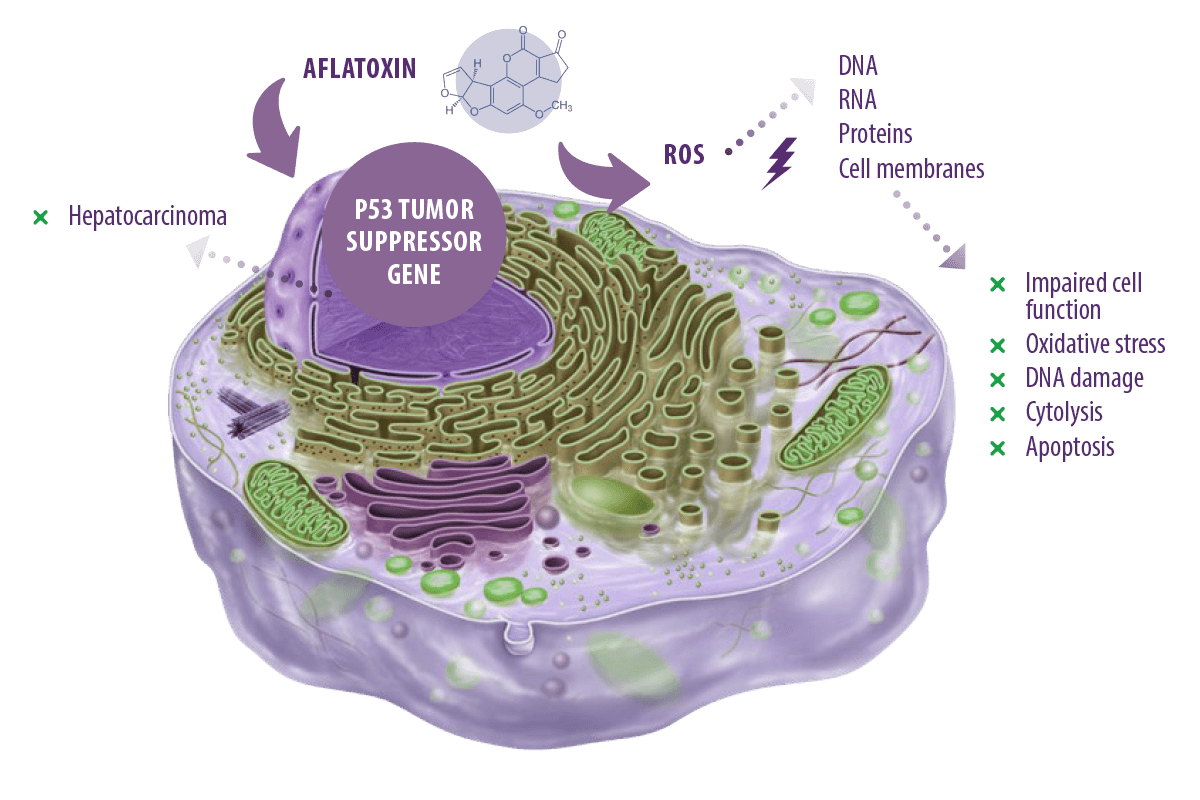
Hepatotoxicity
The metabolism of aflatoxins occurs mainly in the liver under the action of cytochrome P450s into electrophilic, reactive epoxide which, in turn, binds to DNA, RNA, and cellular macromolecules in the liver (Abrar et al., 2013). Aflatoxins are usually associated with the development of hepatocellular carcinoma (HCC) in humans due to their mutagenic and carcinogenic properties.
|
AFB1 is the most potent experimental hepatocarcinogen known (Humans and Cancer, 2002) and no animal model exposed to the toxin has failed to develop HCC.
It also accounts for approximately 9.2 % of all new cancers worldwide (Ferlay et al., 2010). Other liver diseases, including cirrhosis (Humans, 2014) and hepatomegaly (Gong et al., 2012), have also been reported as implications of aflatoxin-induced toxicity in humans.
Levels of serum liver enzymes were changed upon administration of AFB1 through intraperitoneal injection in 5-week-old male Wistar rats. It also increased malondialdehyde (MDA) and decreased glutathione, catalase (CAT), superoxide dismutase (SOD), and glutathione peroxidase (GPx) levels (Ji et al., 2020).
Similar oxidative stress was represented by increased MDA levels and decreasing glutathione content (GSH) and altered liver enzyme levels in rats exposed to AFB1 (Karaca et al., 2021; Vipin et al., 2017).
Nile tilapia (Oreochromis niloticus) exposed to AFB1 suffered from oxidative stress and hepatic damage indicated by liver damage biomarkers represented by decreased CAT activity, GPx, and SOD levels and increased MDA content (Ben Taheur et al., 2022).
LMatrinxã (Brycon cephalus) and Pacu (Piaractus mesopotamicus) showed fatty degeneration, liver damage and altered levels of liver enzymes following dietary exposure to AFB1 (Michelin et al., 2021).
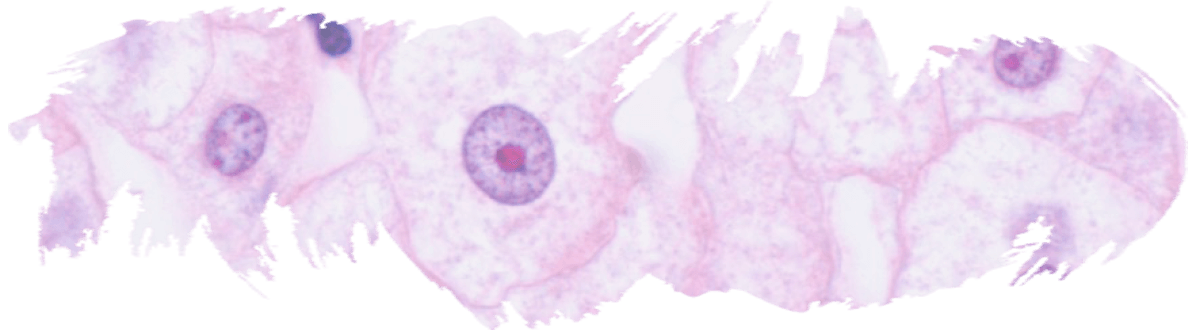
Immunosuppressive effects
The immunosuppressive effects of aflatoxins have been thoroughly investigated in human, poultry, and aquatic species.
AFB1 is toxic to human lymphocytes and its cytotoxicity is mediated by apoptosis and necrosis (Al-Hammadi et al., 2014), and it has been shown to suppress the alternative pathway of complement activation (APCA) in ducks (Valtchev et al., 2015).
Lymphocyte percentage, avian influenza antibody titer relative to thymus weight, and immune response to phytohemagglutinin were decreased in broiler chickens exposed to AFB1 (Rashidi et al., 2020).
Similarly, dietary exposure of broiler chickens to AFB1 induced immune suppressive effects, including a reduction in the immune response to sheep red blood cells (SRBCs), phagocytic clearance of carbon particles, and PHA-P-mediated cutaneous basophilic hypersensitivity, along with degeneration, necrosis, and depletion of lymphoid tissue (Bhatti et al., 2021).
AFB1 reduced bactericidal activity, lysozyme activity, and total serum protein level in yellow catfish (Pelteobagrus fulvidraco) (Wang et al., 2016).
Similarly, lysozyme activities, total immunoglobulin contents, and complement C3 and C4 activities were significantly decreased in the plasma of common carp fed with a diet containing aflatoxins (Bitsayah et al., 2018).
Effects on reproduction
The hepatotoxic and carcinogenic effects of aflatoxins in mammals and aquatic organisms have been intensively reviewed, unlike their effects on reproduction. The mechanism of reproductive toxicity of aflatoxin is not fully understood (Shuaib et al., 2010), but previous studies reported that exposure to aflatoxins induces toxic effects on testis and other reproductive organs with subsequent impairment of spermatogenesis.
It was found that abnormalities in semen parameters (volume, viscosity, pH, fructose, spermatozoa count, morphology, and motility) were evident in infertile men showing highly significant blood and semen aflatoxin levels when compared to their level in fertile men (Uriah et al., 2001).
Varieties of significant changes in reproduction indexes were detected in male Wistar rats injected intramuscularly with 20 μg AFB1/kg body weight (Supriya et al., 2014).
Furthermore, AFB1 was proved to cause pathological changes in the epididymis, such as degeneration and necrosis of epithelial cells of sperm tubules with decreased sperm number (Murad et al., 2015).
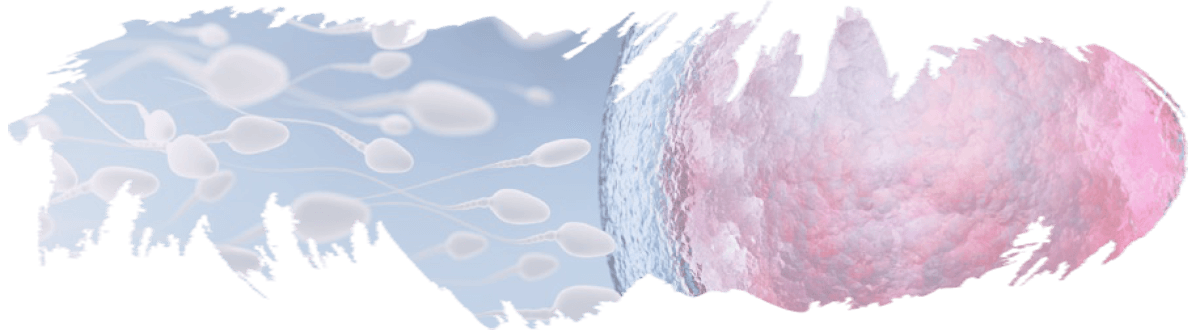
Fumonisins
Fumonisins are produced mainly by Fusarium verticillioides (also known as Fusarium moniliforme), Fusarium proliferatum, Fusarium nygamai, and other Fusarium species, such as Fusarium anthophilum, Fusarium dlamini, Fusarium napiforme, Fusarium subglutinans, Fusarium polyphialidicum and Fusarium oxysporum (Scott, 2012).
There are 16 known types of Fumonisins, referred to as B1 (FB1, FB2, FB3 and FB4), A1, A2, A3, AK1, C1, C3, C4, P1, P2, P3, PH1a and PH1.
They are usually found in corn and corn-based foods (Marasas, 2001).
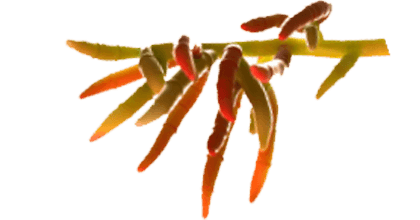
MECHANISMS OF TOXICITY AND EFFECTS
FB1 has a neurotoxic effect in equines as it causes leucoencephalomalacia in horses (Vendruscolo et al., 2016), while its target organ in swine is the lungs as it causes porcine pulmonary edema in pigs (Freitas et al., 2012).
It is carcinogenic, hepatotoxic, nephrotoxic (Szabó et al., 2019; Szabó et al., 2018), and embryotoxic (Lumsangkul et al., 2019) in laboratory animals, while in humans, fumonisins are associated with oesophageal cancer (Yu et al., 2021) and neural tube defects (Copp et al., 2013).
Fumonisins exert their toxic effects mainly by inhibiting the ceramide synthase enzyme, which is essential for the synthesis of ceramide from sphinganine and sphingosine (Voss y Riley, 2013).
Ceramides are the basic structural components of all sphingolipids (found in the cellular membranes of animals and plants) (Engelking, 2015).
⇰ As a result, both sphinganine and sphingosine accumulate following enzyme inhibition causing apoptosis of renal tubule cells and hepatocytes (Voss and Riley, 2013).
It has been further suggested that the pathogenesis of pulmonary edema in swine and cardiotoxicity in horses exposed to FB1 is partly due to inhibition of L-type calcium channels in the heart caused by the accumulated sphingosine and sphinganine, which then leads to left-sided cardiac insufficiency (Voss et al., 2007).
In addition, the carcinogenic effect of fumonisins in humans is related to impaired sphingolipid biosynthesis, which leads to impairment of cellular activity as they are part of cellular membrane composition and essential for cell-cell communication, intracellular and cell-matrix interactions, and growth factors (Edite Bezerra da Rocha et al., 2014).
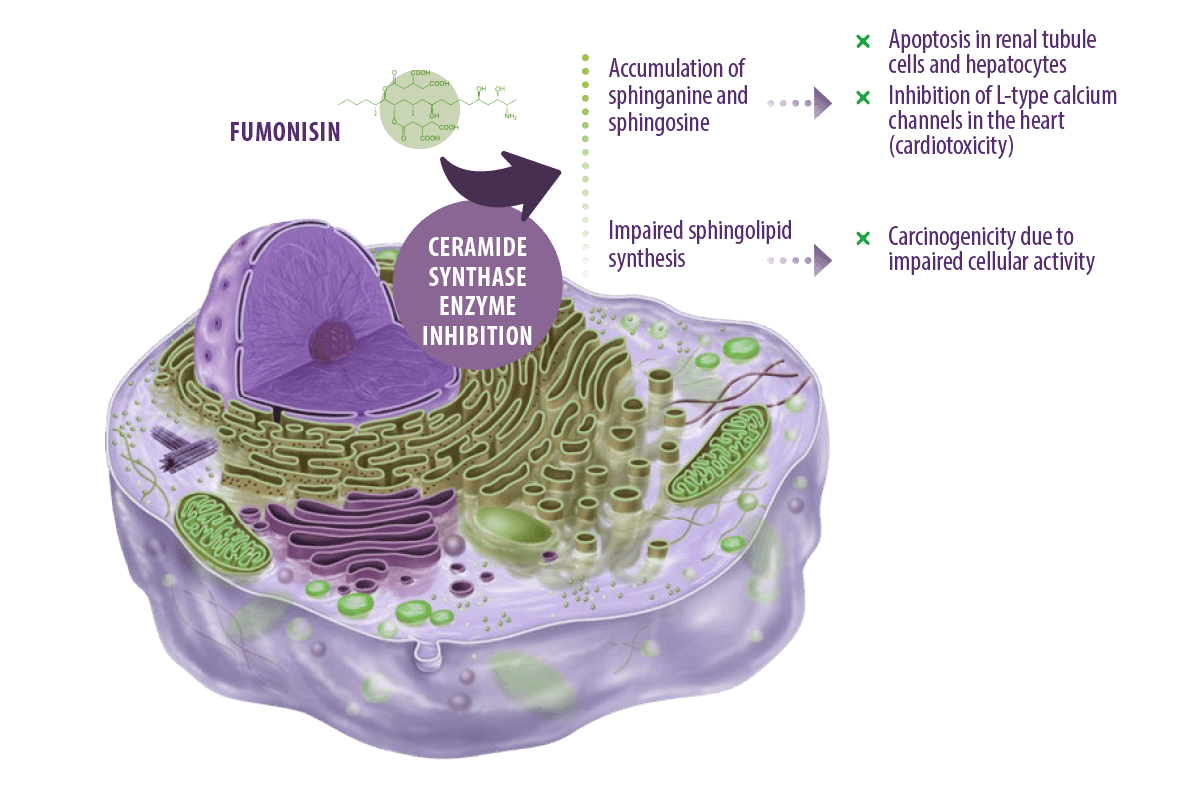
Trichothecenes
Trichothecenes are toxic secondary metabolites produced in host plants, food, and other organic matrices by several fungal genera, including Fusarium, Microcyclospora, Myrothecium, Peltaster, Spicellum, Stachybotrys, Trichoderma, and Trichothecium. Among them, Fusarium trichothecenes are of most significant concern to food and feed safety (Proctor et al., 2018).
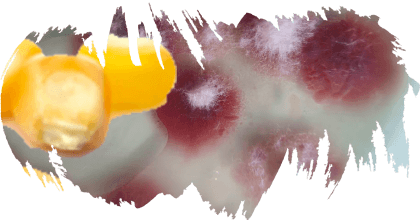
There are more than 150 toxins belonging to the trichothecenes family, but the most important ones are deoxynivalenol (DON), nivalenol (NIV), toxin T2, toxin HT2 and diacetoxyscirpenol (DAS) (Yang et al., 2015).
⇰ Like most mycotoxins, they are a significant food safety concern because of the harmful effects they induce from acute and chronic exposure to them (Sobrova et al., 2010). DON is the most commonly found trichothecene in cereal grains (Tian et al., 2016).
MECHANISMS OF TOXICITY AND EFFECTS
Trichothecenes are known for their capacity to inhibit eukaryotic protein synthesis by binding to the 60S subunit of the eukaryotic ribosomes and inhibiting peptidyl transferase activity, which eventually leads to inhibition of the initiation, elongation, or termination of the chain elongation step in protein synthesis (Arunachalam and Doohan, 2013).
These toxins can also inhibit DNA and RNA synthesis (Minervini et al., 2004), alter cellular membrane structure (Diesing et al., 2011), mitochondrial function, and arrest the cell cycle (Pestka, 2010a).
They can also induce oxidative stress via increasing lipid peroxidation and alteration of antioxidant defenses, which eventually impair protein synthesis and cause DNA damage (Doi and Uetsuka, 2011; Wu et al., 2014).
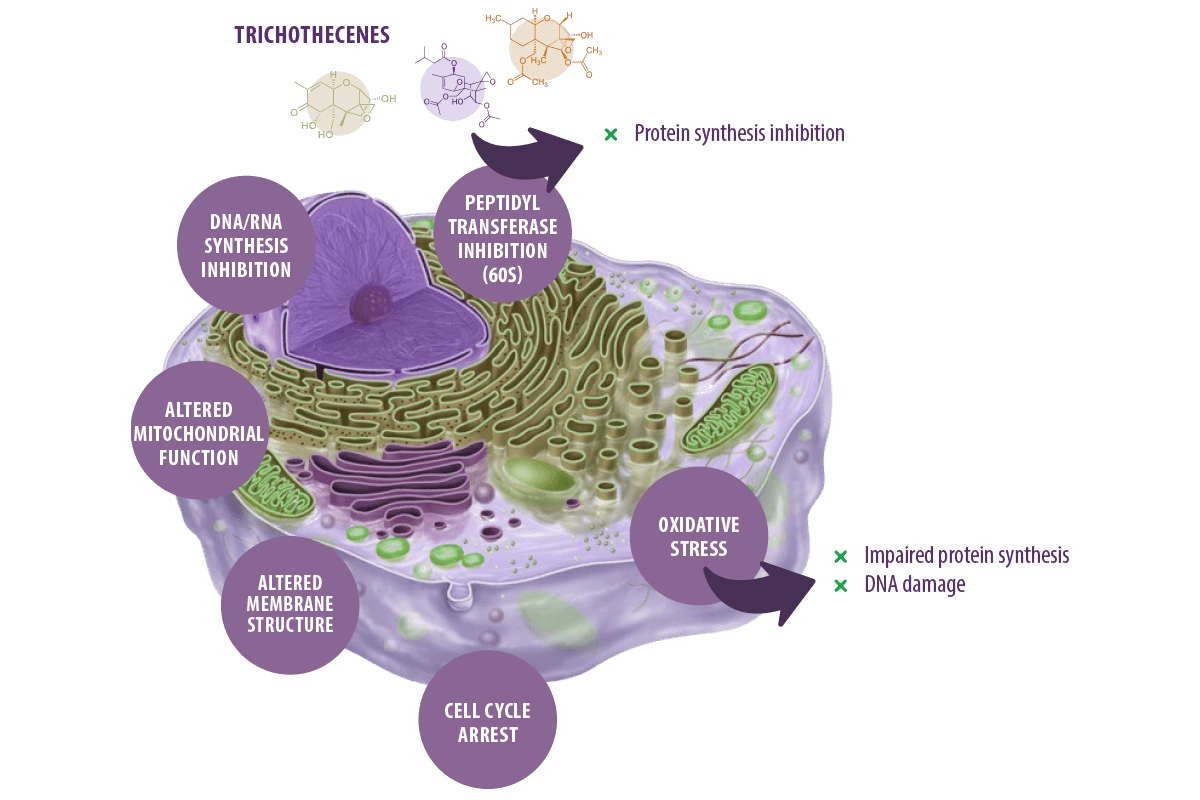
T-2 toxin significantly increased ROS levels, decreased GSH, and elevated lipid peroxidation leading to single-strand breaks in DNA In human cervical cancer cells (Chaudhari et al., 2009).
Elevated concentrations of DON increased ROS levels leading to cell death in a human cell line (Costa et al., 2009).
Dietary exposure of rainbow trout (Oncorhynchus mykiss) to 0.01 mg/ kg b.w. and 0.018 mg/kg b.w T-2 toxin increased lipid peroxidation and the activities of glutathione-S-transferase (GST), glutathione reductase (GR) and glutathione peroxidase (GPx) and decreased in catalase (CAT) activity (Modra et al., 2018).
In the same fish species, significant alterations in activities of GPx in the kidney, GR in the gill and kidney, CAT in the kidney and liver, and GST in the gill and liver followed dietary exposure to DON (Šišperová et al., 2015).
Trichothecenes are well known for inducing apoptosis and programmed cell death (PCD) via ROS- mitochondrial-mediated pathway (Zhuang et al., 2013).
T-2 toxin treatment of ovarian granulose cells of rats caused typical apoptotic morphological changes, such as nuclear fragmentation and reduction in mitochondrial membrane potential, due to the up-regulation of pro-apoptotic proteins p53 and Bax, higher Bax/ Bcl-2 ratio, and the activation of caspase 3 pathway (Wu et al., 2011).
It also induced increased ROS production and cell apoptosis, mainly in the tail areas of zebrafish embryos revealed by Acridine Orange staining (Yuan et al., 2014).
In human chondrocytes, T-2 toxin-induced apoptosis was associated with increased Fas, p53, pro-apoptotic factor Bax, and caspase 3, and downregulation of anti-apoptotic factor Bcl-xl expression (Chen et al., 2008).
Zearalanone
Zearalenone (ZEN), also referred to as the F-2 or RAL mycotoxin, is a non-steroidal estrogenic secondary metabolite biosynthesized mainly by Fusarium graminearum and to a lesser extent by Fusarium culmorum, Fusarium cerealis, or Fusarium equiseti (De Boevre et al., 2012; Taheur et al., 2017).
It has genotoxic (Braicu et al., 2016; Taranu et al., 2015), immunotoxic (Assumaidaee et al., 2020; Hueza et al., 2014), teratogenic, carcinogenic (Abassi et al., 2016), hematotoxic and hepatotoxic properties (Bai et al., 2018) but its toxicity to human and animal arises mainly from its xenosteroidal activities.
Its chemical structure is similar to naturally occurring estrogen and it exerts its action by acting as an endocrine-disrupting compound (EDCs) (Rogowska et al., 2019), potentially changing the functions of the endocrine system in living creatures and causing adverse health effects. In fact, they are known to prompt alterations in hormonally monitored physiological functions (homeostasis, growth, development, and reproduction) (Kar et al., 2021).
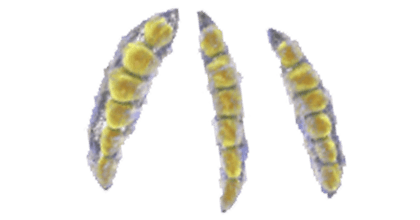
MECHANISMS OF TOXICITY AND EFFECTS
Zearalenone exerts its action by imitating naturally occurring estrogens (leading to a similar outcome as natural estrogens) or competing with their receptors (leading to an antiestrogenic reaction).
⇰ Either way, reproductive impairment is the final outcome (Rogowska et al., 2019).
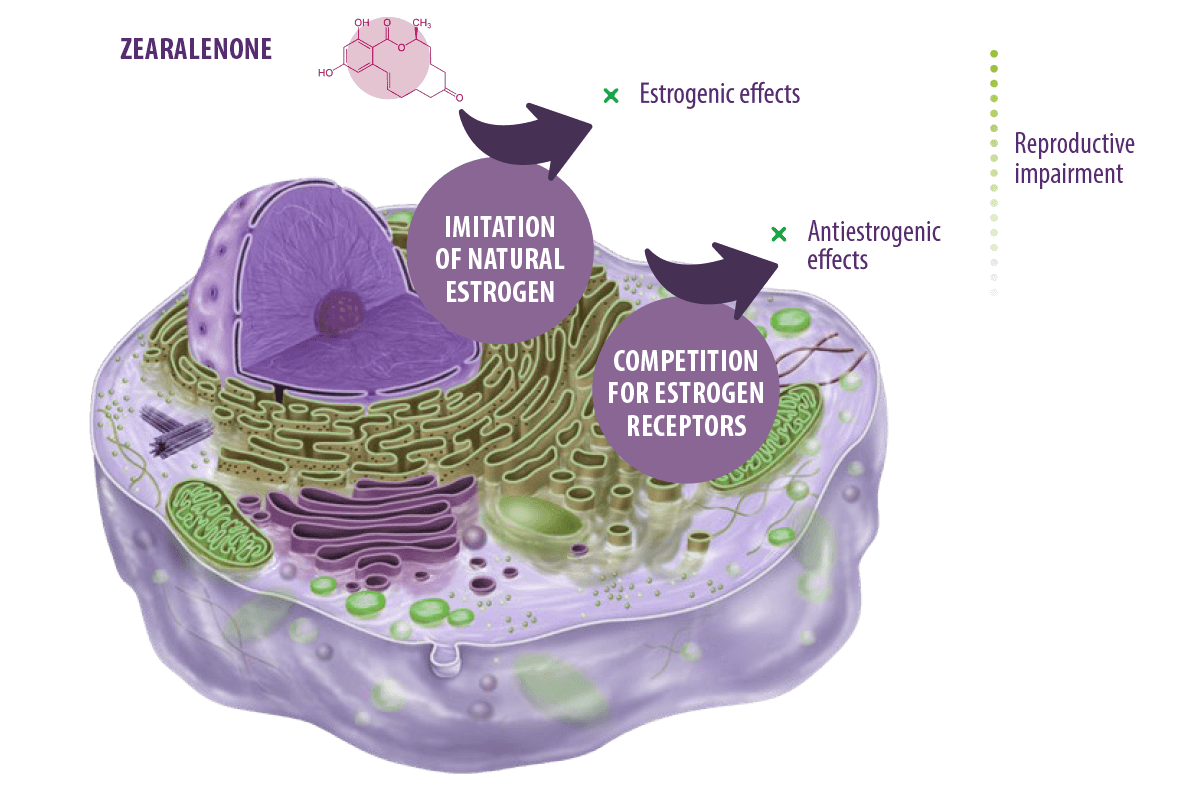
Dietary exposure of piglets to 1mg/kg ZEN induced reproductive impairment represented by an increase in the length, width, and area of the vulva, the genital organ coefficient, and a significant decrease in E2, LH, and FSH (Su et al., 2018).
Similarly, low doses of ZEN affected mice’s male reproductive capacity with a significant decrease in spermatogenic cells, sperm concentration, viability, motility, and hyperactive rate and a significant increase in the DNA double-strand break in spermatogenic cells, in addition to a significant increase in deformity and mortality rate of sperm (Pang et al., 2017).
In fish, several studies reported the effect of zearalenone on reproductive performance, including impaired reproduction of rainbow trout and induced high sperm concentration and high plasma vitellogenin levels in males, and induced early ovarian development in females (Woźny et al., 2020).
In the same line, zearalenone induced vtg-1 mRNA expression in zebrafish (Danio rerio) in a concentration-dependent manner following 120 h exposure (Bakos et al., 2013).
The mechanism of zearalenone-associated immunotoxicity arises because it is a xenoestrogen and EDC.
⇰ Estrogens not only function as reproductive hormones. They also have non-reproductive functions, also affecting immune functions. In this respect, estrogens act on immune cells via estrogen receptors (ERs) which enable them to act either in an immunomodulatory (Islam et al., 2017) or immunosuppressive way (Abbès et al., 2013; Zahran et al., 2021).
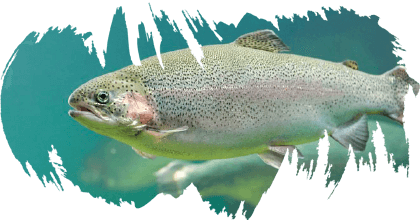
Ochratoxins
Ochratoxins are dihydroisocoumarin bonded to phenylalanine pentaketide metabolites. They are classified as ochratoxin A (OTA), produced by Aspergillus ochraceus, and ochratoxin A, B, and C, produced by other Aspergillus and Penicillium species (Marroquín-Cardona et al., 2014).
Ochratoxins have been found to contaminate various foods like grains, rice, wheat, dried fruit, coffee, cocoa, wine, beer, and foods of animal origin, particularly pork (Kumar et al., 2020).
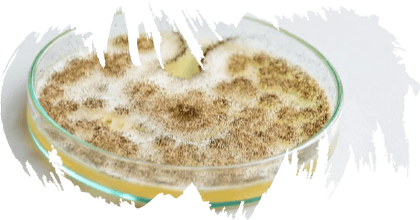
MECHANISMS OF TOXICITY AND EFFECTS
OTA induces toxicity by binding to proteins, particularly serum albumins, with subsequent bioaccumulation in its target organs (Duarte et al., 2012).
It is also an inhibitor of the nuclear factor erythroid 2–related factor 2 (Nrf2), thus inducing physiological oxidative stress, further damaging the DNA (Limonciel and Jennings, 2014).
OTA is mainly nephrotoxic:
- ⇰ In humans, it is responsible for human Balkan endemic nephropathy (BEN) (Stiborová et al., 2016), chronic interstitial nephropathy (CIN) (Hassen et al., 2004), renal failure, and tumors (Chen y Wu, 2017; Hope y Hope, 2012).
- ⇰ In pigs, it causes endemic porcine nephropathy (Jørgensen y Petersen, 2002).
It is also carcinogenic, classified as a Group 2B possible human carcinogen (Marroquín-Cardona et al., 2014).
Ruminants and rodents are, to some extent, resistant to OTA because their microbiota can produce carboxypeptidase enzymes.
⇰ The enzyme can cleave the peptide bond and form less toxic OTα (Abrunhosa et al., 2006).
Despite that fact, OTA is hepatotoxic, teratogenic, immunotoxic, and carcinogenic in experimental models.
It induced hepatotoxicity in rats marked by a significant decrease in SOD, CAT, and GPx activities, a significant increase in MDA level, and histopathological lesions in the liver, including inflammation, steatosis, necrosis, and fibrosis (Damiano et al., 2021).
In another study, OTA exposure was associated with a significant increase in pro-inflammatory and DNA oxidative-damage biomarkers and a significant increase in nitric oxide (NO) levels in kidneys and liver (Longobardi et al., 2021).
The carcinogenic effect of OTA was reported in rats represented by increased mRNA levels of clusterin in kidneys, increased proliferation of cell nuclear antigen (PCNA) in liver and kidney, down-regulation of reactive oxygen species (ROS) and p53 gene, and up-regulation of vimentin and lipocalin in the kidney (Qi et al., 2014).
Furthermore, Liver and kidney impairment was evident in Nile tilapia exposed to OTA (Mansour et al., 2015), while it induced hepatic failure and antioxidative suppression in Thinlip Mullet (Liza ramada) (Magouz et al., 2022).
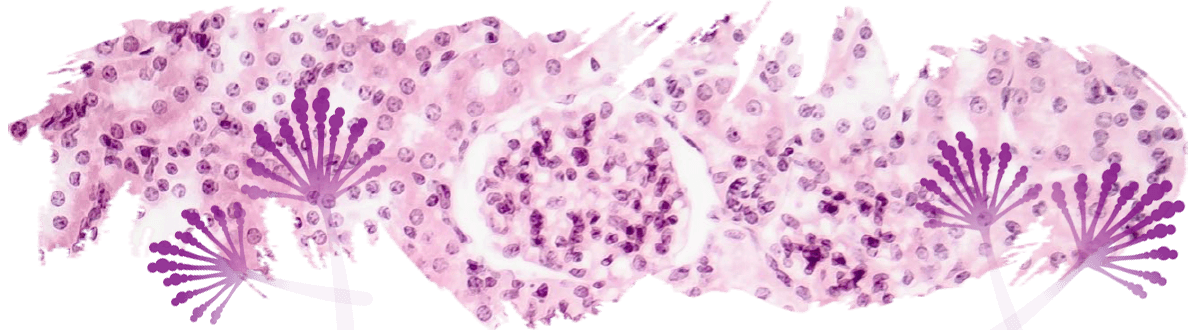
Decontamination of mycotoxins in experimental models
Mycotoxins in feed necessitated the development of novel technologies that would be useful, environmentally friendly, and cost-effective in their removal.
Examples of some compounds used to ameliorate mycotoxin toxicity are described as follows.
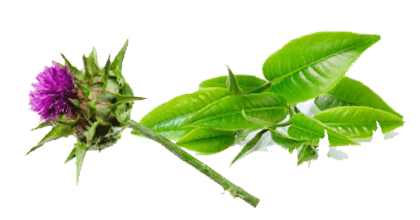
Sodium selenite exhibits protective effects on AFB1-induced toxicity by inhibiting oxidative stress and excessive apoptosis in broilers’ spleen (Wang et al., 2013), decreasing DNA damage and histological alterations in ducklings’ liver (Shi et al., 2015), and ameliorating reproductive toxicity in mice (Cao et al., 2017).
Silymarin administration alleviates elevated Vascular endothelial growth factor (VEGF) and fibroblast growth factor-2 (FGF-2) expression levels and diminishes liver damage induced by FB1 in mice (Sozmen et al., 2014).
Lactobacillus paracasei alleviates genotoxicity, oxidative stress status, and histopathological damage induced by FB1 in mice (Ezdini et al., 2020), while Lb. delbrueckii subsp. lactis (LL) and P. acidilactici (PA) strains induced a protective effect against antigenotoxicity and precancerous lesions caused by FB1 in Sprague-Dawley Rats (Khalil et al., 2015).
Dietary supplementation of rutin in Nile tilapia improves growth, elevated liver antioxidant capacity, and reduced liver and myofiber damage induced by T-2-toxin (Deng et al., 2019).
Oxidative damage and hepatopancreas immune responses induced by T-2 toxin in Chinese mitten crab (Eriocheir sinensis) are reduced following dietary supplementation with arginine (Zhang et al., 2020).
Vitamina C supplementation diminishes reproductive, immune, and hematological toxicity in piglets exposed to ZEN (Su et al., 2018).
Resveratrol (RSV) is able to decrease or reverse ZEN-induced toxicity in adult male Wistar rats, enhancing antioxidant enzyme activity and improving immune parameters in exposed rats (Virk et al., 2020).
Curcumin modulates nitrosative stress, inflammation and DNA damage, hepatotoxicity, and nephrotoxicity induced by Ochratoxin A in Rats (Longobardi et al., 2021).
Green tea-mediated zinc nanoparticles ameliorate the hepatotoxicity and nephrotoxicity induced by OTA in albino rats (Mansour et al., 2015).
Selenium-enriched probiotics (SP) enhance kidney functions, growth performance, and antioxidant parameters in piglets intoxicated with OTA (Gan et al., 2021).
Whey supplementation ameliorates ochratoxicosis in Nile tilapia (Mansour et al., 2015) and dietary Bacillus subtilis protects against hepatic failure, and antioxidative suppression induced by ochratoxin A in Thinlip Mullet (Liza ramada) (Magouz et al., 2022).
The protective role of Minazel® Plus on fish health is evidenced in growth performance, hematological parameters, innate immune and antioxidant responses, bioaccumulation of mycotoxins in liver and musculature, and histopathological assessment of liver and kidney tissues (Zahran et al., 2020).
CONCLUSIONS
Toxigenic secondary fungal metabolites, known as mycotoxins, are regarded as a threat to human health and food safety.
Due to their presence in a wide range of agricultural and food products, they continue to pose a serious threat to the health of animals and humans alike.
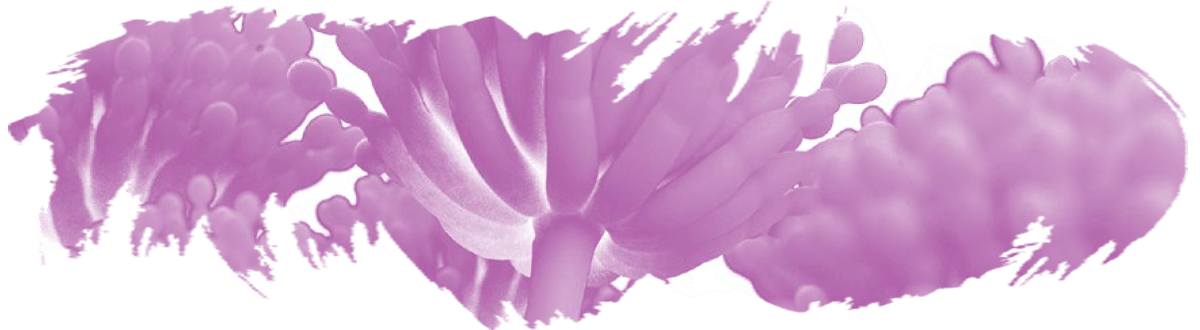
REFERENCES
Abassi, H., Ayed-Boussema, I., Shirley, S., Abid, S., Bacha, H., Micheau, O., 2016. The mycotoxin zearalenone enhances cell proliferation, colony formation and promotes cell migration in the human colon carcinoma cell line HCT116. Toxicology letters 254, 1-7.
Abbès, S., Ben Salah-Abbes, J., Sharafi, H., Oueslati, R., Noghabi, K.A., 2013. Lactobacillus paracasei BEJ01 prevents immunotoxic effects during chronic zearalenone exposure in Balb/c mice. Immunopharmacology and immunotoxicology 35, 341-348.
Abrar, M., Anjum, F.M., Butt, M.S., Pasha, I., Randhawa, M.A., Saeed, F., Waqas, K., 2013. Aflatoxins: biosynthesis, occurrence, toxicity, and remedies. Critical reviews in food science and nutrition 53, 862-874.
Abrunhosa, L., Santos, L., Venâncio, A., 2006. Degradation of ochratoxin A by proteases and by a crude enzyme of Aspergillus niger. Food Biotechnology 20, 231-242.
Al-Hammadi, S., Marzouqi, F., Al-Mansouri, A., Shahin, A., Al-Shamsi, M., Mensah-Brown, E., Souid, A.-K., 2014. The cytotoxicity of aflatoxin b1 in human lymphocytes. Sultan Qaboos Univ Med J 14, e65-e71.
Arunachalam, C., Doohan, F.M., 2013. Trichothecene toxicity in eukaryotes: Cellular and molecular mechanisms in plants and animals. Toxicology letters 217, 149-158.
Asim, M., Sarma, M.P., Thayumanavan, L., Kar, P., 2011. Role of aflatoxin B1 as a risk for primary liver cancer in north Indian population. Clinical biochemistry 44, 1235-1240.
Assumaidaee, A.A., Ali, N.M., Ahmed, S.W., 2020. Zearalenone Mycotoxicosis: Pathophysiology and Immunotoxicity. The Iraqi Journal of Veterinary Medicine 44, 29-38.
Bai, X., Sun, C., Xu, J., Liu, D., Han, Y., Wu, S., Luo, X., 2018. Detoxification of zearalenone from corn oil by adsorption of functionalized GO systems. Applied surface science 430, 198-207.
Bakos, K., Kovács, R., Staszny, Á., Sipos, D.K., Urbányi, B., Müller, F., Csenki, Z., Kovács, B., 2013. Developmental toxicity and estrogenic potency of zearalenone in zebrafish (Danio rerio). Aquatic Toxicology 136-137, 13-21.
Ben Taheur, F., Mansour, C., Mechri, S., Laaouar, H., Safta Skhiri, S., Bouricha, M., Jaouadi, B., Mzoughi, R., Zouari, N., 2022. Protective effects of dietary Kefir against aflatoxin B1‐induced hepatotoxicity in Nile tilapia fish, Oreochromis niloticus. Food Science & Nutrition.
Bhatti, S.A., Khan, M.Z., Saleemi, M.K., Hassan, Z.U., 2021. Combating immunotoxicity of aflatoxin B1 by dietary carbon supplementation in broiler chickens. Environmental Science and Pollution Research 28, 49089-49101.
Bitsayah, A., Banaee, M., Nematdoost Haghi, B., 2018. Effects of aflatoxin-contaminated feed on immunological parameters of common carp (Cyprinus carpio). Iranian Journal of Toxicology 12, 7-12.
Bosetti, C., Turati, F., La Vecchia, C., 2014. Hepatocellular carcinoma epidemiology. Best practice & research Clinical gastroenterology 28, 753-770.
Braicu, C., Cojocneanu-Petric, R., Jurj, A., Gulei, D., Taranu, I., Gras, A.M., Marin, D.E., Berindan-Neagoe, I., 2016. Microarray based gene expression analysis of Sus Scrofa duodenum exposed to zearalenone: significance to human health. BMC genomics 17, 1-10.
Cao, Z., Shao, B., Xu, F., Liu, Y., Li, Y., Zhu, Y., 2017. Protective effect of selenium on aflatoxin B1-induced testicular toxicity in mice. Biological trace element research 180, 233-238.
Carvajal-Moreno, M., 2015. Metabolic changes of aflatoxin B1 to become an active carcinogen and the control of this toxin. Immunome Research 11, 1.
Chaudhari, M., Jayaraj, R., Bhaskar, A., Rao, P.L., 2009. Oxidative stress induction by T-2 toxin causes DNA damage and triggers apoptosis via caspase pathway in human cervical cancer cells. Toxicology 262, 153-161.
Chen, C., Wu, F., 2017. The need to revisit ochratoxin A risk in light of diabetes, obesity, and chronic kidney disease prevalence. Food and Chemical Toxicology 103, 79-85.
Chen, J.-h., Cao, J.-l., Chu, Y.-l., Wang, Z.-l., Yang, Z.-t., Wang, H.-l., 2008. T-2 toxin-induced apoptosis involving Fas, p53, Bcl-xL, Bcl-2, Bax and caspase-3 signaling pathways in human chondrocytes. Journal of Zhejiang University Science B 9, 455-463.
Copp, A.J., Stanier, P., Greene, N.D., 2013. Neural tube defects: recent advances, unsolved questions, and controversies. The Lancet Neurology 12, 799-810.
Costa, S., Schwaiger, S., Cervellati, R., Stuppner, H., Speroni, E., Guerra, M.C., 2009. In vitro evaluation of the chemoprotective action mechanisms of leontopodic acid against aflatoxin B1 and deoxynivalenol-induced cell damage. Journal of Applied Toxicology 29, 7-14.
da Rocha, M.E.B., Freire, F.d.C.O., Maia, F.E.F., Guedes, M.I.F., Rondina, D., 2014. Mycotoxins and their effects on human and animal health. Food Control 36, 159-165.
Damiano, S., Longobardi, C., Andretta, E., Prisco, F., Piegari, G., Squillacioti, C., Montagnaro, S., Pagnini, F., Badino, P., Florio, S., 2021. Antioxidative effects of curcumin on the hepatotoxicity induced by ochratoxin a in rats. Antioxidants 10, 125.
De Boevre, M., Di Mavungu, J.D., Landschoot, S., Audenaert, K., Eeckhout, M., Maene, P., Haesaert, G., De Saeger, S., 2012. Natural occurrence of mycotoxins and their masked forms in food and feed products. World Mycotoxin Journal 5, 207-219.
Deng, Y., Qiu, M., Wang, Y., Wang, R., Lu, P., Sun, L., Li, X., Gooneratne, R., 2019. Protective effect of antioxidant-enriched diets on T-2-toxin-induced damage in tilapia (Oreochromis niloticus). Aquaculture 506, 341-349.
Dhanasekaran, D., Shanmugapriya, S., Thajuddin, N., Panneerselvam, A., 2011. Aflatoxins and aflatoxicosis in human and animals. Aflatoxins-Biochemistry and Molecular Biology 10, 221-254.
Diesing, A.-K., Nossol, C., Dänicke, S., Walk, N., Post, A., Kahlert, S., Rothkötter, H.-J., Kluess, J., 2011. Vulnerability of polarised intestinal porcine epithelial cells to mycotoxin deoxynivalenol depends on the route of application. PloS one 6, e17472.
Doi, A.M., Patterson, P.E., Gallagher, E.P., 2002. Variability in aflatoxin B1–macromolecular binding and relationship to biotransformation enzyme expression in human prenatal and adult liver. Toxicology and applied pharmacology 181, 48-59.
Doi, K., Uetsuka, K., 2011. Mechanisms of mycotoxin-induced neurotoxicity through oxidative stress associated pathways. International journal of molecular sciences 12, 5213-5237.
Duarte, S.C., Lino, C.M., Pena, A., 2012. Food safety implications of ochratoxin A in animal-derived food products. The Veterinary Journal 192, 286-292.
Edite Bezerra da Rocha, M., Freire, F.d.C.O., Erlan Feitosa Maia, F., Izabel Florindo Guedes, M., Rondina, D., 2014. Mycotoxins and their effects on human and animal health. Food Control 36, 159-165.
Engelking, L.R. 2015. Chapter 59 – Sphingolipids, In: Engelking, L.R. (Ed.) Textbook of Veterinary Physiological Chemistry (Third Edition). Academic Press, Boston, 378-383.
Ezdini, K., Ben Salah-Abbès, J., Belgacem, H., Mannai, M., Abbès, S., 2020. Lactobacillus paracasei alleviates genotoxicity, oxidative stress status and histopathological damage induced by Fumonisin B1 in BALB/c mice. Toxicon 185, 46-56.
Ferlay, J., Shin, H.R., Bray, F., Forman, D., Mathers, C., Parkin, D.M., 2010. Estimates of worldwide burden of cancer in 2008: GLOBOCAN 2008. International journal of cancer 127, 2893-2917.
Freitas, B., Mota, M., Del Santo, T., Afonso, E., Silva, C., Utimi, N., Barbosa, L., Vilela, F., Araújo, L., 2012. Mycotoxicosis in Swine: a Review. J An Product Adv 2, 174-181.
Gan, F., Hou, L., Lin, Z., Ge, L., Liu, D., Li, H., Chen, X., Huang, K., 2021. Effects of Selenium-enriched probiotics on ochratoxin A-induced kidney injury and DNMTs expressions in piglets. Research in Veterinary Science 139, 94-101.
Gong, Y.Y., Wilson, S., Mwatha, J.K., Routledge, M.N., Castelino, J.M., Zhao, B., Kimani, G., Kariuki, H.C., Vennervald, B.J., Dunne, D.W., 2012. Aflatoxin exposure may contribute to chronic hepatomegaly in Kenyan school children. Environmental Health Perspectives 120, 893-896.
Hassen, W., Abid, S., Achour, A., Creppy, E., Bacha, H., 2004. Ochratoxin A and β2-microglobulinuria in healthy individuals and in chronic interstitial nephropathy patients in the centre of Tunisia: a hot spot of Ochratoxin A exposure. Toxicology 199, 185-193.
Hope, J.H., Hope, B.E., 2012. A review of the diagnosis and treatment of Ochratoxin A inhalational exposure associated with human illness and kidney disease including focal segmental glomerulosclerosis. Journal of Environmental and Public Health 2012.
Hueza, I.M., Raspantini, P.C.F., Raspantini, L.E.R., Latorre, A.O., Górniak, S.L., 2014. Zearalenone, an estrogenic mycotoxin, is an immunotoxic compound. Toxins 6, 1080-1095.
Humans, I.W.G.o.t.E.o.C.R.t., 2014. DIESEL AND GASOLINE ENGINE EXHAUSTS AND SOME NITROARENES. IARC MONOGRAPHS ON THE EVALUATION OF CARCINOGENIC RISKS TO HUMANS. IARC monographs on the evaluation of carcinogenic risks to humans 105, 9.
Humans, I.W.G.o.t.E.o.C.R.t., Cancer, I.A.f.R.o., 2002. Some traditional herbal medicines, some mycotoxins, naphthalene and styrene, Vol 82. World Health Organization.
Islam, M.R., Kim, J.W., Roh, Y.-S., Kim, J.-H., Han, K.M., Kwon, H.-J., Lim, C.W., Kim, B., 2017. Evaluation of immunomodulatory effects of zearalenone in mice. Journal of Immunotoxicology 14, 125-136.
Jha, A., Krithika, R., Manjeet, D., Verma, R.J., 2013. Protective effect of black tea infusion on aflatoxin induced hepatotoxicity in mice. Journal of Clinical and Experimental Hepatology 3, 29-36.
Ji, Y., Nyamagoud, S.B., SreeHarsha, N., Mishra, A., Gubbiyappa, S.K., Singh, Y., 2020. Sitagliptin protects liver against aflatoxin B1‐induced hepatotoxicity through upregulating Nrf2/ARE/HO‐1 pathway. Biofactors 46, 76-82.
Jørgensen, K., Petersen, A., 2002. Content of ochratoxin A in paired kidney and meat samples from healthy Danish slaughter pigs. Food Additives & Contaminants 19, 562-567.
Kar, S., Sangem, P., Anusha, N., Senthilkumaran, B., 2021. Endocrine disruptors in teleosts: Evaluating environmental risks and biomarkers. Aquaculture and Fisheries 6, 1-26.
Karaca, A., Yilmaz, S., Kaya, E., Altun, S., 2021. The effect of lycopene on hepatotoxicity of aflatoxin B1 in rats. Archives of Physiology and Biochemistry 127, 429-436.
Kew, M.C., 2013. Aflatoxins as a cause of hepatocellular carcinoma. Journal of Gastrointestinal & Liver Diseases 22.
Khalil, A.A., Abou-Gabal, A.E., Abdellatef, A.A., Khalid, A.E., 2015. Protective role of probiotic lactic acid bacteria against dietary fumonisin B1-induced toxicity and DNA-fragmentation in sprague-dawley rats. Preparative Biochemistry and Biotechnology 45, 530-550.
Kumar, P., Mahato, D.K., Sharma, B., Borah, R., Haque, S., Mahmud, M.C., Shah, A.K., Rawal, D., Bora, H., Bui, S., 2020. Ochratoxins in food and feed: Occurrence and its impact on human health and management strategies. Toxicon 187, 151-162.
Li, J., Liu, M. 2019. The carcinogenicity of aflatoxin B1, In: Aflatoxin B1 occurrence, detection and toxicological effects. IntechOpen.
Limonciel, A., Jennings, P., 2014. A review of the evidence that ochratoxin A is an Nrf2 inhibitor: implications for nephrotoxicity and renal carcinogenicity. Toxins 6, 371-379.
Longobardi, C., Damiano, S., Andretta, E., Prisco, F., Russo, V., Pagnini, F., Florio, S., Ciarcia, R., 2021. Curcumin Modulates Nitrosative Stress, Inflammation and DNA Damage and Protects against Ochratoxin A-Induced Hepatotoxicity and Nephrotoxicity in Rats. Antioxidants 10, 1239.
Lu, X., Hu, B., Shao, L., Tian, Y., Jin, T., Jin, Y., Ji, S., Fan, X., 2013. Integrated analysis of transcriptomics and metabonomics profiles in aflatoxin B1-induced hepatotoxicity in rat. Food and chemical toxicology 55, 444-455.
Lumsangkul, C., Chiang, H.-I., Lo, N.-W., Fan, Y.-K., Ju, J.-C., 2019. Developmental toxicity of mycotoxin fumonisin b1 in animal embryogenesis: an overview. Toxins 11, 114.
Magouz, F., Abu-Ghanima, H., Zaineldin, A.I., Gewaily, M.S., Soliman, A., Amer, A.A., Moustafa, E.M., Younis, E.M., Abdel-Warith, A.-W.A., Davies, S.J., Van Doan, H., Tapingkae, W., Dawood, M.A.O., 2022. Dietary Bacillus subtilis relieved the growth retardation, hepatic failure, and antioxidative depression induced by ochratoxin A in Thinlip Mullet (Liza ramada). Aquaculture Reports 22, 100984.
Mansour, T., Omar, A., Soliman, K., Srour, T., Nour, A., 2015. The antagonistic effect of whey on ochratoxin a toxicity on the growth performance, feed utilization, liver and kidney functions of Nile tilapia (Oreochromis niloticus). Middle East J Appl Sci 5, 176-183.
Marasas, W., 2001. Discovery and occurrence of the fumonisins: a historical perspective. Environmental Health Perspectives 109, 239-243.
Marroquín-Cardona, A., Johnson, N., Phillips, T., Hayes, A., 2014. Mycotoxins in a changing global environment–a review. Food and Chemical Toxicology 69, 220-230.
Matejova, I., Svobodova, Z., Vakula, J., Mares, J., Modra, H., 2017. Impact of mycotoxins on aquaculture fish species: a review. Journal of the world aquaculture society 48, 186-200.
Michelin, E., Bedoya-Serna, C., Carrion, L., Levy-Pereira, N., Cury, F., Passarelli, D., Lima, C., Yasui, G., Sousa, R., Fernandes, A., 2021. Effects of dietary aflatoxin on biochemical parameters and histopathology of liver in Matrinxã (Brycon cephalus) and Pacu (Piaractus mesopotamicus) fish. World Mycotoxin Journal 14, 421-430.
Minervini, F., Fornelli, F., Flynn, K., 2004. Toxicity and apoptosis induced by the mycotoxins nivalenol, deoxynivalenol and fumonisin B1 in a human erythroleukemia cell line. Toxicology in Vitro 18, 21-28.
Modra, H., Sisperova, E., Blahova, J., Enevova, V., Fictum, P., Franc, A., Mares, J., Svobodova, Z., 2018. Elevated concentrations of T‐2 toxin cause oxidative stress in the rainbow trout (Oncorhynchus mykiss). Aquaculture Nutrition 24, 842-849.
Murad, A.F., Ahmed, S., Abead, S., 2015. Toxicity effect of aflatoxin B1 on reproductive system of albino male rats. Pakistan Journal of Biological Sciences 18, 107.
Neal, G., Eaton, D., Judah, D., Verma, A., 1998. Metabolism and toxicity of aflatoxins M1and B1in human derivedin VitroSystems. Toxicology and applied pharmacology 151, 152-158.
Pang, J., Zhou, Q., Sun, X., Li, L., Zhou, B., Zeng, F., Zhao, Y., Shen, W., Sun, Z., 2017. Effect of low-dose zearalenone exposure on reproductive capacity of male mice. Toxicology and Applied Pharmacology 333, 60-67.
Patriarca, A., Pinto, V.F., 2017. Prevalence of mycotoxins in foods and decontamination. Current Opinion in Food Science 14, 50-60.
Pestka, J.J., 2010a. Deoxynivalenol-induced proinflammatory gene expression: mechanisms and pathological sequelae. Toxins 2, 1300-1317.
Pestka, J.J., 2010b. Deoxynivalenol: mechanisms of action, human exposure, and toxicological relevance. Archives of toxicology 84, 663-679.
Pinton, P., Tsybulskyy, D., Lucioli, J., Laffitte, J., Callu, P., Lyazhri, F., Grosjean, F., Bracarense, A.P., Kolf Clauw, M., Oswald, I.P., 2012. Toxicity of deoxynivalenol and its acetylated derivatives on the intestine: differential effects on morphology, barrier function, tight junction proteins, and mitogen-activated protein kinases. Toxicological Sciences 130, 180-190.
Proctor, R.H., McCormick, S.P., Kim, H.-S., Cardoza, R.E., Stanley, A.M., Lindo, L., Kelly, A., Brown, D.W., Lee, T., Vaughan, M.M., 2018. Evolution of structural diversity of trichothecenes, a family of toxins produced by plant pathogenic and entomopathogenic fungi. PLoS pathogens 14, e1006946.
Qi, X., Yu, T., Zhu, L., Gao, J., He, X., Huang, K., Luo, Y., Xu, W., 2014. Ochratoxin A induces rat renal carcinogenicity with limited induction of oxidative stress responses. Toxicology and Applied Pharmacology 280, 543-549.
Rashidi, N., Khatibjoo, A., Taherpour, K., Akbari-Gharaei, M., Shirzadi, H., 2020. Effects of licorice extract, probiotic, toxin binder and poultry litter biochar on performance, immune function, blood indices and liver histopathology of broilers exposed to aflatoxin-B1. Poultry Science 99, 5896-5906.
Rogowska, A., Pomastowski, P., Sagandykova, G., Buszewski, B., 2019. Zearalenone and its metabolites: Effect on human health, metabolism and neutralisation methods. Toxicon 162, 46-56.
Rotimi, O.A., Rotimi, S.O., Duru, C.U., Ebebeinwe, O.J., Abiodun, A.O., Oyeniyi, B.O., Faduyile, F.A., 2017. Acute aflatoxin B1–Induced hepatotoxicity alters gene expression and disrupts lipid and lipoprotein metabolism in rats. Toxicology reports 4, 408-414.
Scott, P., 2012. Recent research on fumonisins: a review. Food additives & contaminants: part A 29, 242 248.
Sforza, S., Dall’Asta, C., Marchelli, R., 2006. Recent advances in mycotoxin determination in food and feed by hyphenated chromatographic techniques/mass spectrometry. Mass Spectrometry Reviews 25, 54-76.
Shi, D., Liao, S., Guo, S., Li, H., Yang, M., Tang, Z., 2015. Protective effects of selenium on aflatoxin B1 induced mitochondrial permeability transition, DNA damage, and histological alterations in duckling liver. Biological trace element research 163, 162-168.
Shuaib, F.M., Ehiri, J., Abdullahi, A., Williams, J.H., Jolly, P.E., 2010. Reproductive health effects of aflatoxins: a review of the literature. Reproductive Toxicology 29, 262-270.
Šišperová, E., Modrá, H., Ziková, A., Kloas, W., Blahová, J., Matejová, I., Živná, D., Svobodová, Z., 2015. The effect of mycotoxin deoxynivalenol (DON) on the oxidative stress markers in rainbow trout (Oncorhynchus mykiss, Walbaum 1792). Journal of Applied Ichthyology 31, 855-861.
Sobrova, P., Adam, V., Vasatkova, A., Beklova, M., Zeman, L., Kizek, R., 2010. Deoxynivalenol and its toxicity. Interdisciplinary toxicology 3, 94.
Sozmen, M., Devrim, A.K., Tunca, R., Bayezit, M., Dag, S., Essiz, D., 2014. Protective effects of silymarin on fumonisin B1-induced hepatotoxicity in mice. Journal of veterinary science 15, 51-60.
Stiborová, M., Arlt, V.M., Schmeiser, H.H., 2016. Balkan endemic nephropathy: an update on its aetiology. Archives of toxicology 90, 2595-2615.
Streit, E., Schatzmayr, G., Tassis, P., Tzika, E., Marin, D., Taranu, I., Tabuc, C., Nicolau, A., Aprodu, I., Puel, O., 2012. Current situation of mycotoxin contamination and co-occurrence in animal feed—Focus on Europe. Toxins 4, 788-809.
Su, Y., Sun, Y., Ju, D., Chang, S., Shi, B., Shan, A., 2018. The detoxification effect of vitamin C on zearalenone toxicity in piglets. Ecotoxicology and Environmental Safety 158, 284-292.
Supriya, C., Girish, B., Reddy, P.S., 2014. Aflatoxin B1-induced reproductive toxicity in male rats: possible mechanism of action. International journal of toxicology 33, 155-161.
Szabó, A., Fébel, H., Ali, O., Kovács, M., 2019. Fumonisin B1 induced compositional modifications of the renal and hepatic membrane lipids in rats–Dose and exposure time dependence. Food Additives & Contaminants: Part A 36, 1722-1739.
Szabó, A., Szabó-Fodor, J., Kachlek, M., Mézes, M., Balogh, K., Glávits, R., Ali, O., Zeebone, Y.Y., Kovács, M., 2018. Dose and exposure time-dependent renal and hepatic effects of intraperitoneally administered fumonisin B1 in rats. Toxins 10, 465.
Taheur, F.B., Fedhila, K., Chaieb, K., Kouidhi, B., Bakhrouf, A., Abrunhosa, L., 2017. Adsorption of aflatoxin B1, zearalenone and ochratoxin A by microorganisms isolated from Kefir grains. International journal of food microbiology 251, 1-7.
Taranu, I., Braicu, C., Marin, D.E., Pistol, G.C., Motiu, M., Balacescu, L., Neagoe, I.B., Burlacu, R., 2015. Exposure to zearalenone mycotoxin alters in vitro porcine intestinal epithelial cells by differential gene expression. Toxicology Letters 232, 310-325.
Tian, Y., Tan, Y., Liu, N., Liao, Y., Sun, C., Wang, S., Wu, A., 2016. Functional agents to biologically control deoxynivalenol contamination in cereal grains. Frontiers in microbiology 7, 395.
Uriah, N., Ibeh, I.N., Oluwafemi, F., 2001. A study on the impact of aflatoxin on human reproduction. African Journal of Reproductive Health, 106-110.
Valtchev, I., Koynarski, T., Sotirov, L., Nikolov, Y., Petkov, P., 2015. Effect of Aflatoxin B1 on Moulard Duck’s Natural Immunity. Pakistan Veterinary Journal 35.
Varga, J., Frisvad, J.C., Samson, R., 2011. Two new aflatoxin producing species, and an overview of Aspergillus section Flavi. Studies in mycology 69, 57-80.
Vendruscolo, C.P., Frias, N.C., De Carvalho, C., De Sá, L., Belli, C.B., Baccarin, R.Y.A., 2016. Leukoencephalomalacia outbreak in horses due to consumption of contaminated hay. Journal of veterinary internal medicine 30, 1879.
Vila-Donat, P., Marín, S., Sanchis, V., Ramos, A., 2018. A review of the mycotoxin adsorbing agents, with an emphasis on their multi-binding capacity, for animal feed decontamination. Food and chemical toxicology 114, 246-259.
Vipin, A., Rao, R., Kurrey, N.K., KA, A.A., Venkateswaran, G., 2017. Protective effects of phenolics rich extract of ginger against Aflatoxin B1-induced oxidative stress and hepatotoxicity. Biomedicine & pharmacotherapy 91, 415-424.
Virk, P., Al-Mukhaizeem, N.A.R., Morebah, S.H.B., Fouad, D., Elobeid, M., 2020. Protective effect of resveratrol against toxicity induced by the mycotoxin, zearalenone in a rat model. Food and Chemical Toxicology 146, 111840.
Voss, K., Smith, G., Haschek, W., 2007. Fumonisins: Toxicokinetics, mechanism of action and toxicity. Animal feed science and technology 137, 299-325.
Voss, K.A., Riley, R.T., 2013. Fumonisin toxicity and mechanism of action: Overview and current perspectives. Food Safety 1, 2013006-2013006. Wang, F., Shu, G., Peng, X., Fang, J., Chen, K., Cui, H.,
Chen, Z., Zuo, Z., Deng, J., Geng, Y., 2013. Protective effects of sodium selenite against aflatoxin B1-induced oxidative stress and apoptosis in broiler spleen. International journal of environmental research and public health 10, 2834-2844.
Wang, X., Wang, Y., Li, Y., Huang, M., Gao, Y., Xue, X., Zhang, H., Encarnação, P., Santos, G.A., Gonçalves, R.A., 2016. Response of yellow catfish (Pelteobagrus fulvidraco) to different dietary concentrations of aflatoxin B1 and evaluation of an aflatoxin binder in offsetting its negative effects. Ciencias marinas 42, 15 29.
Woźny, M., Dobosz, S., Hliwa, P., Gomułka, P., Król, J., Obremski, K., Blahova, J., Svobodova, Z., Michalik, O., Ocalewicz, K., 2020. Feed-borne exposure to zearalenone impairs reproduction of rainbow trout. Aquaculture 528, 735522.
Wu, J., Jing, L., Yuan, H., Peng, S.-q., 2011. T-2 toxin induces apoptosis in ovarian granulosa cells of rats through reactive oxygen species-mediated mitochondrial pathway. Toxicology letters 202, 168-177.
Wu, Q.-H., Wang, X., Yang, W., Nüssler, A.K., Xiong, L.-Y., Kuča, K., Dohnal, V., Zhang, X.-J., Yuan, Z.-H., 2014. Oxidative stress-mediated cytotoxicity and metabolism of T-2 toxin and deoxynivalenol in animals and humans: an update. Archives of toxicology 88, 1309-1326.
Yang, S., Wang, Y., Beier, R.C., Zhang, H., Ruyck, K.D., Sun, F., Cao, X., Shen, J., Zhang, S., Wang, Z., 2015. Simultaneous determination of type A and B trichothecenes and their main metabolites in food animal tissues by ultraperformance liquid chromatography coupled with triple-quadrupole mass spectrometry. Journal of agricultural and food chemistry 63, 8592-8600.
Yang, X., Lv, Y., Huang, K., Luo, Y., Xu, W., 2016. Zinc inhibits aflatoxin B1-induced cytotoxicity and genotoxicity in human hepatocytes (HepG2 cells). Food and Chemical Toxicology 92, 17-25.
Yu, S., Jia, B., Liu, N., Yu, D., Zhang, S., Wu, A., 2021. Fumonisin B1 triggers carcinogenesis via HDAC/PI3K/Akt signalling pathway in human esophageal epithelial cells. Science of The Total Environment 787, 147405.
Yuan, G., Wang, Y., Yuan, X., Zhang, T., Zhao, J., Huang, L., Peng, S., 2014. T-2 toxin induces developmental toxicity and apoptosis in zebrafish embryos. Journal of Environmental Sciences 26, 917-925.
Zahran, E., Elbahnaswy, S., Mamdouh, A.Z., El‐Matbouli, M., 2021. Xenosteroids in aquaculture with special consideration to Lake Manzala (Northern delta lake, Egypt): Types, sources and mechanism of action. Aquaculture Research 52, 5962-5977.
Zahran, E., Risha, E., Hamed, M., Ibrahim, T., Palić, D., 2020. Dietary mycotoxicosis prevention with modified zeolite (Clinoptilolite) feed additive in Nile tilapia (Oreochromis niloticus). Aquaculture 515.
Zain, M.E., 2011. Impact of mycotoxins on humans and animals. Journal of Saudi chemical society 15, 129 144.
Zhang, C., Chi, C., Liu, J., Ye, M., Zheng, X., Zhang, D., Liu, W., 2020. Protective effects of dietary arginine against oxidative damage and hepatopancreas immune responses induced by T-2 toxin in Chinese mitten crab (Eriocheir sinensis). Fish & Shellfish Immunology 104, 447-456.
Zhuang, Z., Yang, D., Huang, Y., Wang, S., 2013. Study on the apoptosis mechanism induced by T-2 toxin. PloS one 8, e83105.