R. K. Asrani y Rakesh Kumar
Department of Veterinary Pathology, Dr G C Negi College of Veterinary and Animal Sciences, CSK Himachal Pradesh Agricultural University, Palampur, Himachal Pradesh, India
Mycotoxins are secondary harmful mold metabolites that produce significant detrimental health effects in human beings and animals1. These are low molecular weight compounds known to be harmful even at low concentrations2.

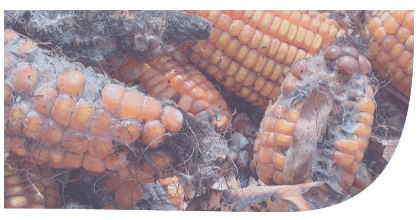
The most frequently encountered harmful mycotoxins in foodstuffs and feed include aflatoxin B1 (AFB1), ochratoxin A (OTA), trichothecenes, HT-2 and T-2 toxins, fumonisin B1 (FB1), citrinin (CTN), zearalenone (ZEN) and ergot alkaloids.
⇰ A predominately marked distribution of fumonisins, zearalenone and deoxynivalenol (DON) is documented globally4.
Cereal crops may become contaminated in the field or during harvesting, transport, processing or storage5,6. The rate of contamination of crops with fungus is more frequently triggered by the rainy season7.
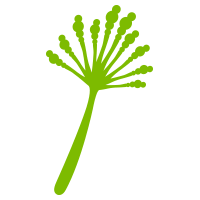
Factors facilitating the production of mycotoxins in contaminated products include8:
- ⇰ Moisture content (20-25%)
- ⇰ Environmental temperature (22-30ºC)
- ⇰ Composition of food items
- ⇰ Relative air humidity (70-90%)
- ⇰ Physical damage to cereals by pests
- ⇰ pH
- ⇰ Presence of mold spores
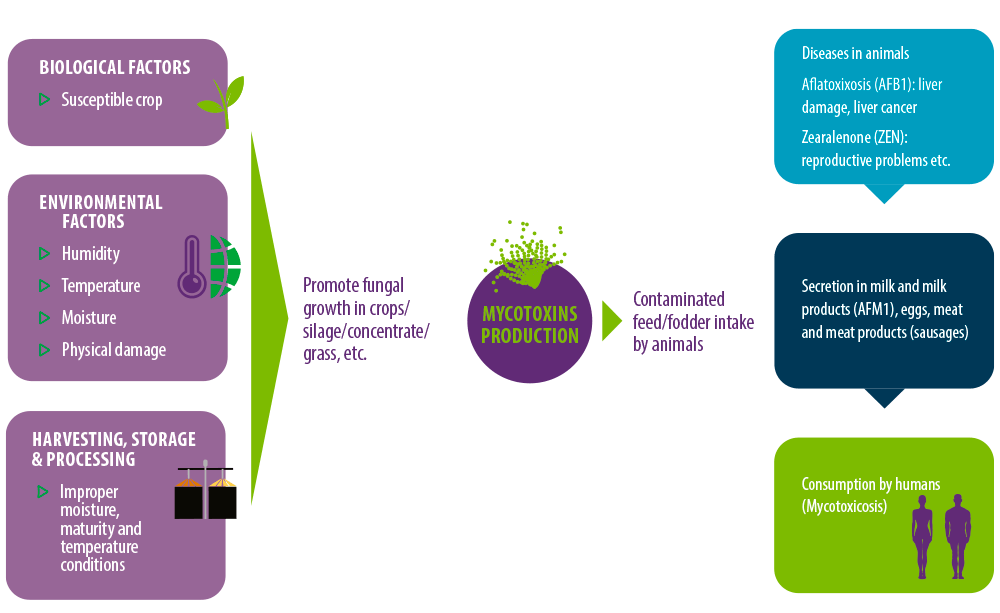
Figure 1. Mycotoxins production and their occurrence in the food chain.
Common routes of entry of mycotoxins into the body are:
⇰ Direct consumption of contaminated products of plant origin (cereals, nuts, bread etc.) and products obtained from animals (meat and meat products, milk, offal’s, fermented sausages etc.)9,10.
⇰ Dermal contact and inhalation are not very common routes but can act as a potential mode of entry into the body11.
Harmful toxic effects of mycotoxins depend on11,12:
Type of mycotoxin
Dose introduced into the body
Duration of exposure to the mycotoxins
Mycotoxins are known to produce several harmful effects in animals and human beings. Classification of these toxins can be made on the basis of toxicity13 and clinical symptoms related to the organs damaged14.