Al-Zahraa Mamdouh1 and Eman Zahran2*
1Fish Diseases Department National Institute of Oceanography and Fisheries, Egypt
2Department of Internal Medicine, Infectious and Fish Diseases, Faculty of Veterinary Medicine, Mansoura University, Egypt
*Correspondence: [email protected]
The aquaculture industry is considered an important sector for providing aquatic products for the increasing human consumption of animal food across the world (Ahmad et al., 2021).
Commercial compound feed is used as aquafeed to provide the necessary nutrients for growth and maintaining farmed fish health in aquaculture (Oliveira y Vasconcelos, 2020).
Commercial aquafeed is usually a mixture of products of plant and animal origin (Oliveira y Vasconcelos, 2020).
- ⇰ Soybean meal and other cereals are used as plant-based ingredients in aquafeed preparation (Matejova et al., 2017b).
- ⇰ Fish meal and fish oil are products of marine origin used mainly in processing aquafeed as they are important sources of proteins, vital amino acids, and long-chain polyunsaturated fatty acids (Jackson, 2012).
Increased demand for aquaculture production has resulted in an increased demand for fish meal and fish oil used in aquafeed. Subsequently, the shortage of pelagic fish and other fish species used in production is now an ecologic and economic implication (Oliveira y Vasconcelos, 2020).
Therefore, there has been a recent tendency to use ingredients of plant origin as an alternative to fish meal and fish oil.
Soy products are plant-based ingredients used as substitutes for fishmeal.
Soybean meal is the most common ingredient used in fish feed as an alternative to fishmeal. It usually constitutes 15-45% of aquafeed for herbivorous and omnivorous fish (Tacon et al., 2011).
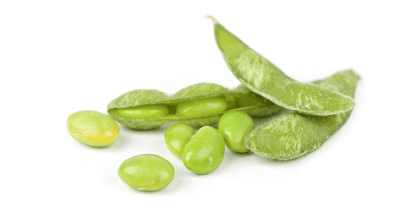
Recently, plant-based aquafeed has been used in a wider range worldwide. However, fish feed of plant origin is subjected to infection with various fungi and contamination with mycotoxins (Deng et al., 2021; Mwihia et al., 2020; Pietsch, 2020).
The occurrence of mycotoxins in commercial aquafeed has been a widespread problem (Gonçalves et al., 2018; Marijani et al., 2017).
Mycotoxins are secondary metabolites produced by a group of filamentous fungi that infect a wide variety of food and feedstuff all over the world (Juraschek et al., 2022).
They are produced by fungal strains belonging mainly to the genus Aspergillus, Fusarium, Penicillium, Claviceps, or Alternaria (Edite Bezerra da Rocha et al., 2014) and contaminate many crops, including maize, rice, nuts, corn, wheat, soybean, and sorghum either before, during harvest or processing and storage of feed.
Contamination of food and feedstuff with mycotoxins deteriorates the nutritional value and represents a potential risk for human, animal, and fish health (da Rocha et al., 2014; Koletsi et al., 2021; Omotayo et al., 2019).
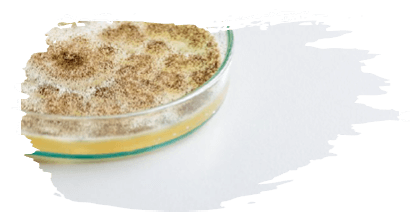
Common mycotoxin types in fish feeds
Aflatoxins (AFs) were first detected in black tiger shrimp (Penaeus monodon) feed in the Philippines (Bautista et al., 1994).
Later, these mycotoxins were detected in some fish feeds from fish farming processes in Thailand (Altuğ y Özyurt, 2003) and in commercial shrimp feed
in Turkey (Bintvihok et al., 2003).
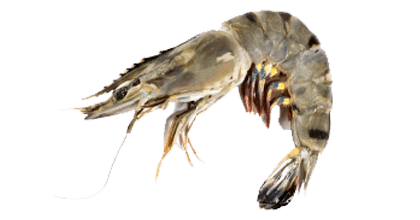
Shrimp and fish feed samples were contaminated with zearalenone and ochratoxin A (OTA) in Thailand while in India, shrimp feed was contaminated with AFs (Fegan y Spring, 2007).
Zearalenone (ZEN) was detected in trout feed collected from two farms in northeastern Poland (Woźny et al., 2013), while Pietsch et al. (2013) detected the presence of deoxynivalenol (DON) in common carp (Cyprinus carpio) feed samples from central Europe.
Nácher-Mestre et al. (2015) reported the occurrence of Fumonisins (FUM) and DON in Atlantic salmon (Salmo salar) and gilthead sea bream (Sparus aurata) feeds.
In Argentina, AFs, OTA, T-2 toxin, DON, and ZEN were detected in salmonid feeds (Greco et al., 2015).
In Africa, Nile tilapia (Oreochromis niloticus) and African catfish (Clarias gariepinus) feeds from Kisumu, Kenya, Kigembe, Rwanda, Jinja, Uganda were found to be highly contaminated with AF, FUM, and DON (Marijani et al., 2017).
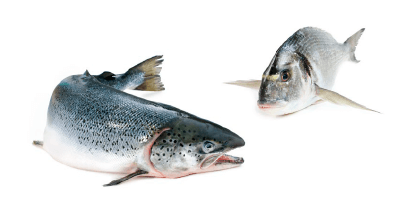
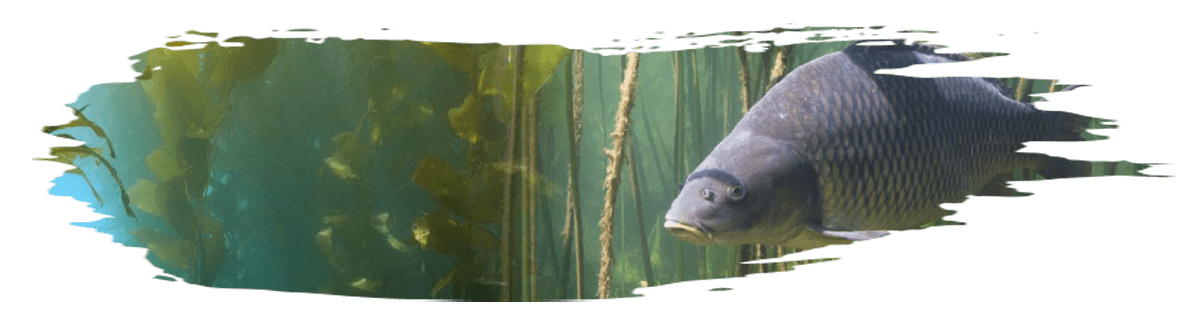
Aflatoxins
Aflatoxins are produced mainly by Aspergillus flavus and Aspergillus parasiticus and to a minor extent by Aspergillus nomius, Aspergillus bombycis, Aspergillus pseudotamari, and Aspergillus ochraceoroseus (Varga et al., 2011).
⇰ AFB1, B2, G1 and G2 are the main aflatoxins and they are classified according to their blue or green fluorescence under ultraviolet light (Dhanasekaran et al., 2011). They are mycotoxins are produced in a variety of feedstuffs, including maize, ground nuts, rice and sorghum cottonseed, spices, cereals, soybean, cocoa, and meat (Patriarca and Pinto, 2017; Vila-Donat et al., 2018).
Aflatoxins induce their toxicity by reacting with cellular macromolecules, including nucleic acids, proteins, and phospholipids, and induce various genetic, metabolic, signaling, and cell structure disruptions (Rushing and Selim, 2017).
In addition, they impair cell function and integrity through the induction of oxidative stress (Ayala et al., 2014). Moreover, they induce genotoxicity and immunotoxicity by acting on genomic DNA, other functional macromolecules, and immunocompetent cells (Benkerroum, 2020).
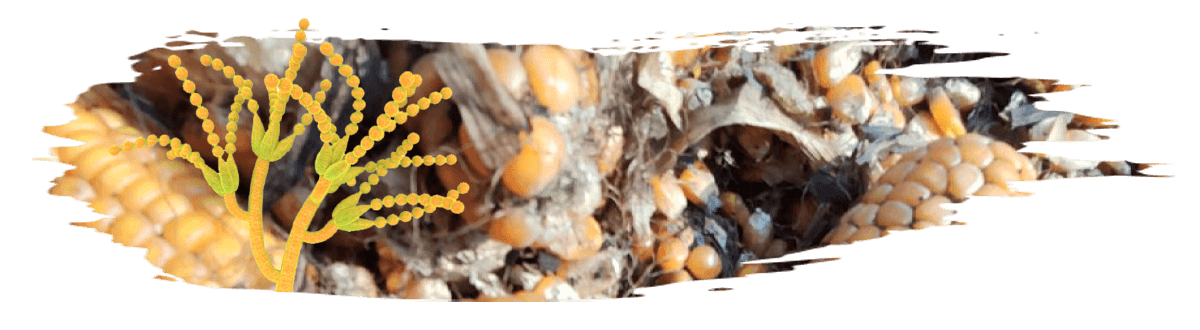
Fumonisins
Fumonisins are produced mainly by Fusarium verticillioides (also known as Fusarium moniliforme), Fusarium proliferatum, and Fusarium nygamai.
⇰ There are 16 known types of fumonisins with FB1 being the most found in food and feedstuff such as rice, sorghum, beer, triticale, cowpea seeds, beans, soybeans, and asparagus (Scott, 2012).
The mechanism of toxicity of fumonisins arises from the fact that it has a similar structure to sphingolipids (sphingosine and sphinganine) which are essential to synthesize ceramide under the action of ceramide synthase (Chen et al., 2021).
Fumonisins, particularly FB, can inhibit ceramide synthase by competing with sphingolipids leading to the accumulation of sphinganine and sphingosine, causing apoptosis of renal tubule cells and hepatocytes (Voss y Riley, 2013).
Fumonisins can also induce oxidative stress and immune toxicity in humans (Wang et al., 2016), animals (Chen et al., 2021), and different fish species (Baldissera et al., 2020b; Lala et al., 2021).
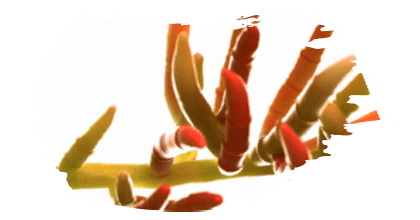
Trichothecenes
Trichothecenes are produced by various fungal genera including Fusarium, Microcyclospora, Myrothecium, Peltaster, Spicellum, Stachybotrys, Trichoderma, and Trichothecium (Proctor et al., 2018).
⇰ There are more than 150 toxins of trichothecenes but the most studied ones are deoxynivalenol (DON) and toxin T2 (Yang et al., 2015).
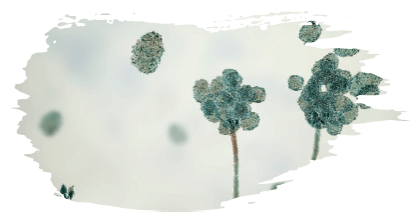
The toxic mechanisms associated with trichothecenes, including T-2 toxin and DON, are related to their effects on protein synthesis as they bind to the 60S subunit of eukaryotic ribosomes and inhibit peptidyl transferase activity which eventually inhibits the initiation, elongation, or termination of the chain elongation step in protein synthesis (Arunachalam y Doohan, 2013).
This results in the upregulation of c-Jun N-terminal kinase (JNK)/p38 mitogen-activated protein kinase (p38 MAPK) (Li et al., 2022).
In addition, T-2 toxin can:
Inhibit the activation of the cyclic adenosine monophosphate (cAMP) pathway.
Decrease the production of steroidogenic acute regulatory protein (StAR).
Decrease the synthesis of the steroid hormones, estradiol and progesterone.
- (Wu et al., 2015)
Downregulate growth hormone receptor (GHR) by inhibiting the suppressor of cytokine signaling (SOCS)-related mechanisms and signal transducers (Amuzie and Pestka, 2010) and by activating signal transducer and activator of transcription (STAT)-related mechanisms (Wang et al., 2012).
Zearalenone
Zearalenone (ZEN), F-2, or RAL is produced mainly by Fusarium graminearum and to a minor extent by Fusarium culmorum, Fusarium cerealis, and Fusarium equiseti (De Boevre et al., 2012; Taheur et al., 2017).
Zearalenone is a xenoestrogen and sex-steroid hormone mimic (a type of xenohormone that imitates estrogen) (Zahran et al., 2021).
Chemically, its structure is similar to natural estrogen and it is considered an endocrine-disrupting compound (EDCs) (Rogowska et al., 2019) that can potentially alter the functions of the endocrine system and cause alterations in physiological functions monitored hormonally including homeostasis, growth, development, and reproduction (Kar et al., 2021).
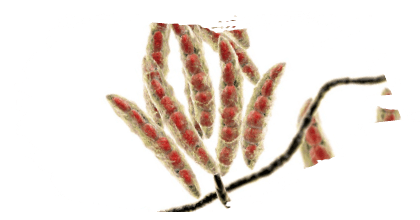
Ochratoxins
Ochratoxins are dihydroisocoumarin bonded to phenylalanine pentaketide metabolites produced by A. ochraceus that produce ochratoxin A (OTA) and by other Aspergillus and Penicillium species that produce ochratoxin A, B and C (Marroquín-Cardona et al., 2014).
⇰ OTA is the most prevalent type in food and feed worldwide and it is the most studied form of all ochratoxins in experimental models, including fish.
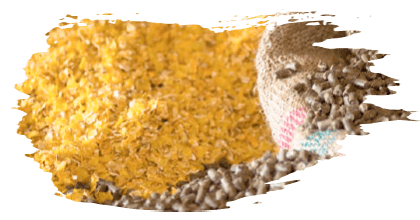
It induces toxicity through different pathways that can be conducive to oxidative stress and increased reactive oxygen species (ROS) via the inhibition of activation and gene transcription of nuclear factor erythroid 2–related factor 2 (Nrf2) leading to lipid peroxidation, proteotoxic stress and oxidative DNA damage (Limonciel y Jennings, 2014).
In addition, it is associated with the disruption of calcium homeostasis, leading to a sustained increase in the cytosolic calcium level and cytotoxicity (Rahimtula y Chong, 1991).
Moreover, one of the main mechanisms of its toxicity is the inhibition of protein synthesis resulting in the slowing of cell growth or proliferation and impairment of cell metabolism (Creppy et al., 1983).
OTA is mainly nephrotoxic, as it is responsible for human Balkan endemic nephropathy (BEN) (Stiborová et al., 2016), chronic interstitial nephropathy (CIN) (Hassen et al., 2004) and renal failure and tumors (Chen and Wu, 2017; Hope and Hope, 2012) whereas in pigs it can cause endemic porcine nephropathy (Jørgensen and Petersen, 2002).
It is also hepatotoxic (Longobardi et al., 2021; Shin et al., 2019), teratogenic (Stoev, 2022), immune-toxic (Gan et al., 2022), and carcinogenic (classified as a Group 2B, possible human carcinogen) (Marroquín-Cardona et al., 2014).
Toxic effects of mycotoxins on fish health
Mycotoxins affect the growth, reproduction, and other physiological functions and health status of fish.
⇰ They have a significant impact on weight gain, body composition, biochemical functions, and the general health condition of fish.
Many studies have discussed the effect of different mycotoxins on the growth, reproduction, and immune state of fish.
Mycotoxins impair growth and induce oxidative stress in fish
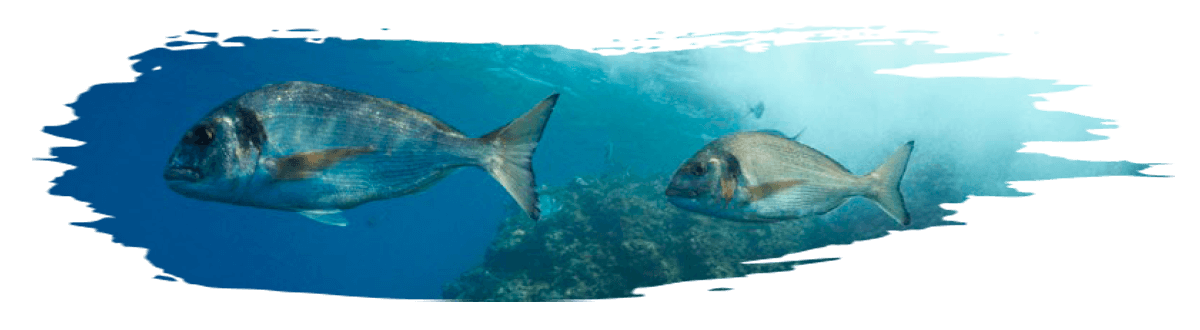
AFLATOXINS
Aflatoxins are the most studied mycotoxins affecting growth and liver functions in fish.
They are known to affect protein synthesis and cellular integrity of the liver, where the generation of intracellular reactive oxygen species such as superoxide anion, hydroxyl radical, and hydrogen peroxide (H2O2) is a result of the metabolism of aflatoxins in the liver under the action of cytochrome P450 (Asim et al., 2011).
⇰ These compounds target cellular DNA, RNA, proteins, and cell membranes, leading to the impairment of cell functioning, DNA damage, and oxidative stress (Abrar et al., 2013).
Many studies have examined the impact of aflatoxins on growth and liver functions in different fish species.
Nile tilapia fed a diet contaminated with 2mg/kg diet AFB1 for 14 weeks showed significantly reduced final body weight, weight gain, feed conversion, total protein, albumin, and globulin levels and significantly increased serum alanine transaminase (ALT) and aspartate transaminase (AST) (Ayyat et al., 2018).
The same fish species fed a diet containing 2.5 mg AFB1/kg displayed a state of oxidative stress manifested by significantly reduced final weight, weight gain, specific growth rate, total feed intake, feed conversion ratio, and protein efficiency ratio, as well as significantly reduced serum catalase (CAT) and glutathione (GSH) concentrations.
In addition, the liver showed several pathological signs, including necrosis of pancreatic acini with pyknotic and karyorrhectic nuclei, as well as hydropic degeneration with fatty change and early necrotic changes in most hepatocytes with congestion, hemorrhage, and lymphocytic infiltration (Naiel et al., 2019).
Feeding Nile tilapia with a diet contaminated with a mix of aflatoxins (AFs; AFB1, AFB2, AFG1, AFG2) at a level of 16 μg/kg increased lipid peroxidation and suppressed the antioxidation system where it significantly increased malondialdehyde (MDA) content and significantly decreased CAT, superoxide dismutase (SOD) activity and GSH level (Zahran et al., 2020).
Rainbow trout (Oncorhynchus mykiss) fed a diet containing 50 ppb AFB1 for 30 days showed significantly decreased final weight, weight gain, specific growth rate, and feed conversion ratio (Ghafarifarsani et al., 2021).
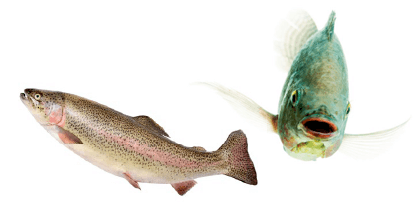
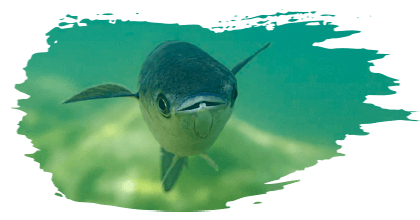
Similarly, dietary 1.0 mg/kg AFB1 affected growth and caused oxidative stress to Sea Bass (Lateolabrax maculatus) as it reduced final body weight, weight gain rate, specific growth rate, feed intake, condition factor, viscerosomatic index, hepatosomatic index while increasing the levels of serum total antioxidant capacity (TAOC), SOD, CAT, MDA, alkaline phosphatase (AKP) (Peng et al., 2021).
In a more recent study, diets supplemented with aflatoxin B1 at 1 mg/kg impaired growth performance, hepatorenal functions, and antioxidative capacity of Grey Mullet (Liza ramada) where it significantly reduced final weight, weight gain, specific growth rate, feed conversion ratio, total serum protein, albumin, and globulin levels awhile it significantly increased ALT and AST (Khalafalla et al., 2022).
FUMONISINS
The inclusion of 50 mg FB/kg feed in the diet of Nile tilapia fingerlings affected their growth by altering the hepatic mRNA expression of growth hormone receptor (GHR) and insulin-like growth factor (IGF-1) genes ) (da Silva et al., 2019).
AIn addition, Supplementation of a diet containing 10.0 mg FB1/kg for 56 days was conducive to significantly reduced final weight, weight gain, specific growth rate, and feed conversion ratio in catfish (Heterobranchus longifilis) juveniles (Adeyemo et al., 2016b), and catfish (Clarias gariepinus) (Adeyemo et al., 2016a).
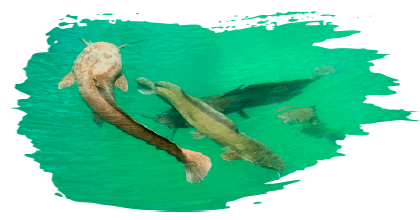
TRICHOTHECENES
Growth impairment and oxidative stress have been reported in many studies as consequences of trichothecenes toxicity.
Rainbow trout (O. mykiss) displayed a significant decrease in feed intake, weight gain, growth rate, and feed efficiency when fed diets contaminated with 2.6 ppm of (Hooft et al., 2011) and 2.1ppm (Hooft et al., 2019) DON for 8 weeks.
Red tilapia (O. niloticus × O. mossambicus) fed wheat naturally contaminated with 41 mg/kg DON for 8 weeks showed decreased growth rate, feed intake, and feed efficiency (Tola et al., 2015).
Oral exposure of Carp to DON resulted in the down-regulation of biotransformation enzymes, activation of alanine aminotransferase, and reduction of several enzymes involved in glutathione cycling and reduction of oxidative stress, alongside histopathological lesions in liver tissue, including fat aggregation, vacuolization, and hyperemia (Modra et al., 2018).
Feeding Rainbow Trout (O. mykiss) with a diet containing 1.8 mg T2 toxin /kg feed induced oxidative stress in the liver manifested by increasing glutathione-S-transferase (GST), glutathione reductase (GR) and glutathione peroxidase (GPx) and decreasing CAT activity and impaired liver function which was evident by decreasing serum levels of total protein, albumin, and alkaline phosphatase (ALP) enzyme (Pietsch y Burkhardt-Holm, 2015).
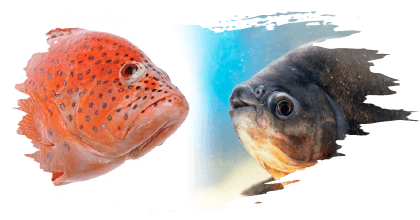
OCHRATOXINS
Ochratoxins (particularly OTA) have been reported extensively to induce the same effects on fish growth and the antioxidant system. For example, the growth performance and muscle antioxidant system of Tambaqui (Colossoma macropomum) juveniles were impaired when fed a diet contaminated with 1.6 mg ochratoxin A (OTA)/kg diet for 14 days where weight gain, daily weight gain, specific growth rate, and feed efficiency percent were significantly reduced while muscle ROS and lipid peroxidation (LPO) was significantly increased (Baldissera et al., 2020c).
In the same line, Nile tilapia fed a diet containing 80 μg OTA/kg fish suffered from growth impairment and alteration of liver function where final weight, weight gain, and specific growth rate were significantly reduced, while glutamic-oxaloacetic transaminase (GOT) and ALP were significantly increased (Mansour et al., 2015).
In addition, OTA at a level of 4 or 8 mg OTA/kg diet for 56 days affected the growth performance of Channel Catfish (Ictalurus punctatus) by significantly decreasing feed intake and impairing the feed conversion ratio (Zahran et al., 2016).
Feed-borne exposure of Grass Carp (Ctenopharyngodon idella) to 2406 µg of OTA/kg of diet-induced growth retardation evident by significantly decreased feed efficiency, percentage weight gain, and specific growth rate (Liu et al., 2020).
In a more recent study, OTA diet supplementation at a dose of 1 mg/Kg for 8 weeks induced growth retardation, hepatic failure, and antioxidative depression in Thinlip Mullet (L. ramada), where OTA significantly reduced final body weight, weight gain, specific growth rate, serum total protein, albumin, and globulin levels while significantly increased liver function tests AST and ALT. Furthermore, it significantly decreased CAT, SOD, and GPx activities and significantly increased MDA content (Magouz et al., 2022).
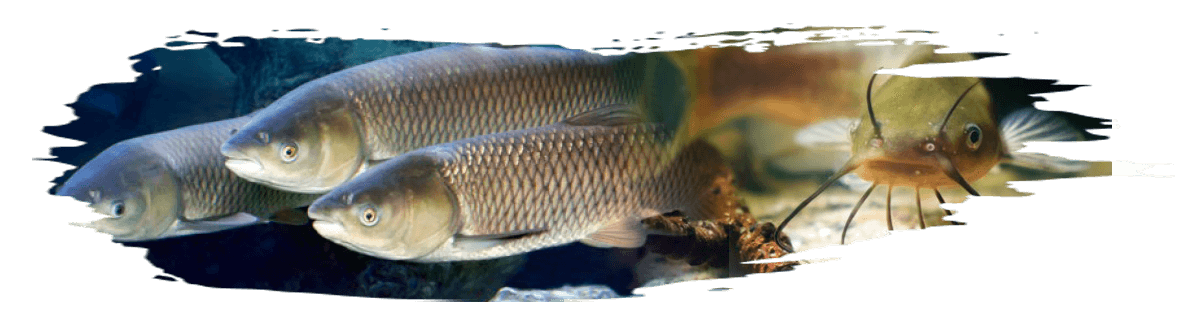
ZEARALENONE
The effect of zearalenone on fish growth is variable where it can either promotes or impair growth, probably depending on its dose and fish species.
Rainbow Trout fed on a diet containing ZEN at a dose of 2 mg/kg feed resulted in higher feeding efficiency and growth rate (Woźny et al., 2019).
Growth parameters such as condition factor, hepatosomatic index, gonadosomatic index and caspase 3 activity were not significantly altered in Zebrafish (Danio rerio) fed diet containing 10 µg ZEN/L for 21 days (Muthulakshmi et al., 2018a).
The growth parameters of Goldfish (Carassius auratus) including weight gain, specific growth rate, feed conversion ratio, and body protein were not significantly altered after feeding on a diet containing 500 ZEN ppb for 60 days (Hassanpour et al., 2021).
Feed-borne exposure to ZEN (0.725 g/kg diet) for 14 days impaired the growth performance of European Sea Bass (Dicentrarchus labrax) by significantly decreasing final weight, weight gain, and specific growth rate (Abdel-Tawwab et al., 2020).
Although the first response of fish to zearalenone exposure, as any environmental contaminant, is the induction of oxidative stress, few studies have been reported to study its effect on the antioxidant system. However, oxidative stress was induced in Zebrafish (D. rerio) embryos after feeding on a diet containing 950 µg/L ZEN where it significantly increased ROS production and LPO and significantly decreased CAT, SOD, GPx activities and GST and GSH levels (Muthulakshmi et al., 2018b).
In a more recent study, European Sea Bass (D. labrax) exposed to ZEN (0.725 g/kg diet) for 4 weeks suffered from oxidative stress manifested by significantly decreased SOD, CAT, GPx activities and significantly increased MDA content (Abdel-Tawwab et al., 2021).
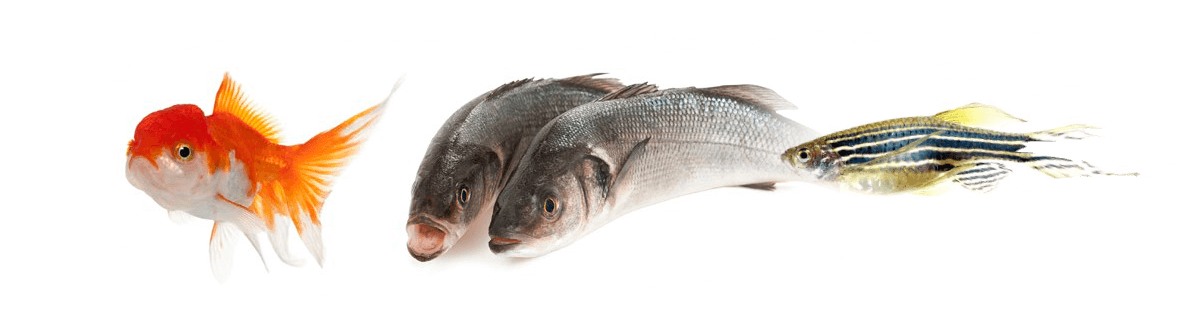
The effects of mycotoxins on fish immunity
Almost all mycotoxins supplemented in fish diets in experimental models induced immunotoxicity.
Immunity biomarkers, including hematological profiles and innate and adaptive immune responses, were extensively studied in fish to demonstrate their immune responses against toxicity induced by different types of mycotoxins.
AFLATOXINS
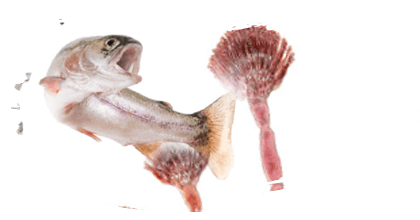
Aflatoxins induce immunotoxicity by impairing immunocompetent cells, affecting the production of immune response mediators, and disrupting innate and adaptive immunity (Benkerroum, 2020).
Complement (C3; COMP3) was significantly reduced when Nile Tilapia was fed a diet containing 2.5 mg AFB1/kg (Naiel et al., 2019).
The same fish species displayed reduced antibody titer against the S. agalactiae vaccine when fed a diet containing 3 ppm AFB1 for 6 weeks (Pumipuntu et al., 2013).
The expression of tumor necrosis factor-alpha (TNFα) and interleukin-8 (IL8) genes were significantly up-regulated in Rainbow Trout (O. mykiss) following feeding on die (Ghafarifarsani et al., 2021).
The expression of interleukin1β (IL1β) and transforming growth factor β (TGFβ) were downregulated in Nile Tilapia fed a diet containing 200 ppb for 16 weeks (Mahfouz, 2015).
Chinese Sea Bass (Lateolabrax maculatus) fed a diet supplemented with 1mg/kg food AFB1 displayed an immune impairment manifested by increased serum lysozyme activity, liver lysozyme activity, and immunoglobulin M (IgM) level (Peng et al., 2021).
Nile Tilapia fed diet contaminated with 2mg/kg diet AFB1 for 14 weeks suffered from impaired hematological and immune responses manifested by significantly reduced red blood cell (RBCs), hemoglobin (Hb) content, and increased white blood cells (WBCs) count (Ayyat et al., 2018).
Nile Tilapia fed diet contaminated with a mix of aflatoxins (AFs; AFB1 and 2, AFG1 and 2) at a dose of 16 μg/kg suffered from immunosuppression manifested by a significant decrease in RBCs, Hb, and packed cell volume (PCV) and changes in dynamics of leukocyte counts (specifically neutrophils), in addition to a significant decrease in serum lysozyme and bactericidal activity (Zahran et al., 2020).
In a more recent study, diets supplemented with aflatoxin B1 at 1 mg/kg impaired the hematological profile of Grey Mullet (L. ramada) where it significantly reduced Hb, RBCs, and WBCs count (Khalafalla et al., 2022).
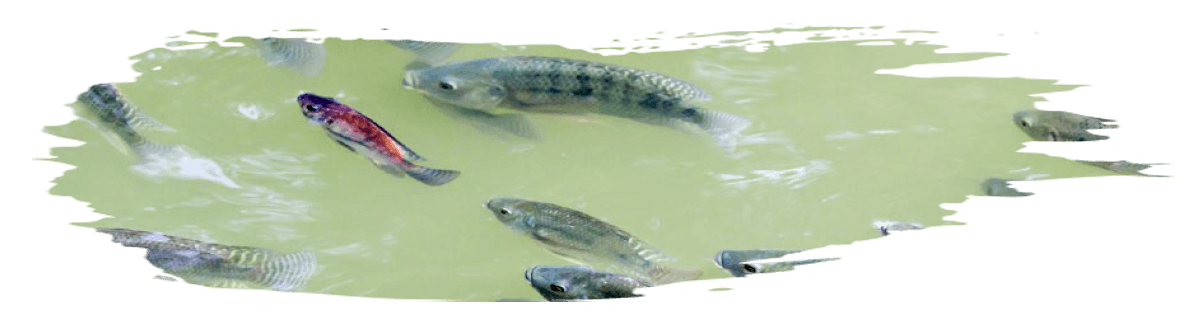
ZEARALENONE
Zearalenone affects the immune responses of fish by mimicking the action of endogenous sex steroids on estrogen receptors (ERS) in immune cells.
⇰ ERS have been identified in many immune components in different fish species allowing a pathway for xenoesteroids to induce their effects and influence immune functions and causing either immune stimulation or immune suppression (Zahran et al., 2021).
ZEN contamination at a dose of 0.725 mg/kg in the diet of European Sea Bass for 4 weeks induced macrocytic hypochromic anaemia with significant reduced values of RBCs, Hb, hematocrit (Ht), and mean corpuscular hemoglobin concentration (MCHC) together with significant elevations in mean corpuscular volume (MCV) and mean corpuscular hemoglobin (MCH) values.
Likewise, lymphopenia, monocytosis, leucopenia, eosinophilia, neutrophilia, and thrombocytopenia with a drastic reduction in WBC counts, in addition to a significant reduction of serum lysozyme activity have been reported (Abdel-Tawwab et al., 2020).
In another study with similar fish species and similar experimental conditions, ZEN induced a significant decline in total serum immunoglobulins, significant down-regulation of interleukin-4 (IL-4) and interleukin 1 beta (IL-1β) genes, and significant up-regulation of tumor necrosis factor-alpha (TNF-α) and heat shock protein 70 (HSP70) genes in the liver and anterior kidney (Abdel-Tawwab et al., 2021).
Zearalenone induced immuno-compromised status via the TOR/NF/κB pathway and aggravated the spread of Aeromonas hydrophila to Grass Carp gut (Ctenopharyngodon idella) where exposure to 2507 μg ZEN/kg diet for 70 days reduced the transcription of β-defensin-1, Hepcidin, liver expressed antimicrobial peptide 2A/2B (LEAP-2A/2B) and Mucin-2, this resulted in the invasion of the intestinal barrier by bacteria, which was shown by activation of the nuclear factor κB (NF-κB) and the expression of downstream cytokines (Zhang et al., 2021).
Zearalenone may have tissue-specific immune properties where feed-borne exposure of Rainbow Trout to 2mg ZEN/Kg feed for 72 weeks induced immunomodulatory responses in the spleen and liver with significant increase in the expression of IL-4, IL-17, and interferon gamma (IFNy) while it had immunosuppressive effect in the kidney with expression of IL-17 and IFNγ significantly decreased. It also enhanced innate and adaptive immune responses with significantly increased blood lymphocyte and thrombocytes counts (Woźny et al., 2019).
In an experiment with juvenile Carp, fish exposed to ZEN at doses of 0.62 and 0.80 mg/ kg feed for 4 weeks had significantly increased granulocytes and decreased monocytes in their blood. Further analysis of leukocytes isolated from the head and the trunk kidney of these fish revealed that exposure to the low and the medium dose of ZEN (0.33 and 0.62 mg/kg) increased the respiratory burst of the immune cells, whereas the higher dose (0.80 mg/kg) decreased this response (Pietsch et al., 2015b).
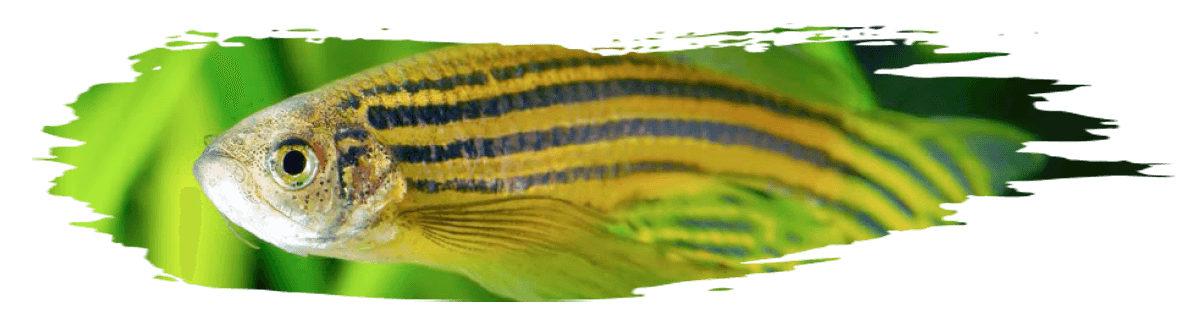
FUMONISINS
Fumonisins, particularly FB1, are also reported to induce immune-toxicity in fish where dietary exposure of Silver Catfish to 6.2 mg/kg feed FB1 for 30 days generated a pro-inflammatory profile that contributed to impairment of immune and inflammatory responses, via reduction of splenic triphosphate diphosphohydrolase (NTPDase) activity and thrombocytes counts in total blood (Baldissera et al., 2020a).
Clarid Fish (H. longifilis) fed s diet containing 40mg/Kg FB1 for 56 days manifested a significantly increased erythrocyte count and significantly decreased Hb concentration and Leucocytes count (Adeyemo et al., 2016b).
FB1, in combination with ZEN at a sublethal dose, exerted a toxic effect on immune and antioxidant responses on Zebrafish embryos where the activities of CAT, caspase-3, and detoxification enzyme cytochromes P450 (CYP450), as well as the expressions of six genes (Mn-sod, cas9, bax, cc-chem, ERα , and crh) associated with oxidative stress, cellular apoptosis, immune system, and endocrine system were significantly altered (Yang et al., 2021).
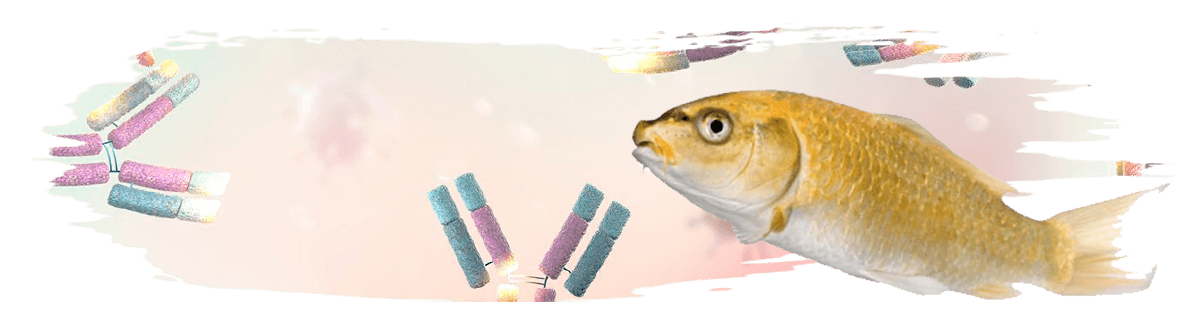
TRICHOTHECENES
Trichothecenes are also reported to be involved in impairing the immune responses in fish.
Common carp (C. carpio L) suffered from anaemia, leukopenia and impaired immune responses when fed diet containing 5.3 mg/kg T-2 toxin for a month, where it decreased Hb, RBC, PCV and WBC, including lymphocytes and neutrophils, phagocyte oxidative burst and total immunoglobulins level and significantly increased complement activation (Matejova et al., 2017a).
Rainbow Trout (O. mykiss) exposed to 1.8 mg/kg T2 toxin for 28 days manifested a significantly decreased Hb concentration and bactericidal activity and significantly increased erythrocyte count, total leucocytic and lymphocytic counts (Modra et al., 2020).
Feed-borne exposure of Carp (Cyprinus carpio L) to DON at a dose of 953 μg/kg feed for 56 days altered the innate immune responses where it significantly increased the expression of pro-inflammatory (ifnγ, tnfα-2, il-8, inos) and anti-inflammatory (il-10, arg-1, and arg-2) immune-related genes in different organs including head kidney, trunk kidney, spleen, liver, and intestine at different times after the exposure. In addition, sub-chronic exposure (26 days of DON treatment) resulted in the activation of arginases, culminating in increased arginase activity in head kidney leukocytes (Pietsch et al., 2015a).
OCHRATOXINS
Various studies have investigated the immunotoxicity of ochratoxins, mainly OTA, in different fish species.
OTA exposure at a dose of 2.4 mg/kg caused immune cell proliferation in the spleen of Atlantic Salmon (S. salar) proved by a significant increase in mRNA expression of two immune markers, IFNγ and Ki67 (Bernhoft et al., 2018).
Exposure at a dose of 8 mg OTA/kg diet increased susceptibility of Channel Catfish (I. punctatus) to experimental water mold Saprolegnia (Zahran et al., 2016). The hematologic profile of Nile Tilapia was altered following feed-borne exposure to 600 μg OTA/kg diet which significantly decreased RBCs, Hb, Hct, MCV, MCH, and MCHC (Shalaby y Abbassa, 2009).
Feeding on a diet containing 80 and 160 μg OTA/kg BW caused innate and adaptive immunosuppression in Nile tilapia manifested by a significant reduction in WBCs, lymphocytes, phagocytic activity, phagocytic index, alongside a significant dose-dependent reduction in antibody titers against vaccination with A. hydrophila bacterin (Mansour et al., 2016).
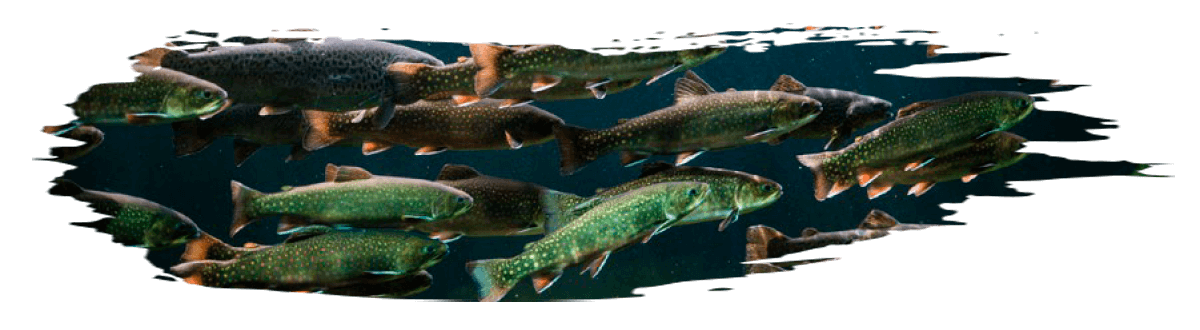
The effects of mycotoxins on fish reproduction
Zearalenone is the most common and potent mycotoxin affecting fish reproduction due to its xenosteroidal nature.
Many studies reported the toxic effect of zearalenone on the reproductive system of Zebrafish (D. rerio) as an experimental model. Female zebrafish exposed to 10 μg/L zearalenone for 21 days displayed a significant decline in the gonadosomatic parameters, an increase in caspase 3 activities and vitellogenin (Vtg) expression, and significant upregulation of estrogen receptor α (Erα), Vtg, luteinizing hormone receptor, steroidogenic acute regulatory protein, 3β-HSD, 17β- HSD, CYP19a1 and CYP19a1b genes. In addition to oocyte atresia and oocyte membrane detachment in ovaries (Muthulakshmi et al., 2018a).
Zearalenone induced vtg-1 mRNA expression in a concentration-dependent manner following 120 h exposure (Bakos et al., 2013).
Exposure of the same fish to 1000 ng ZEN/L for 21 days reduced relative spawning frequency and relative fecundity and increased plasma VTG level in males (Schwartz et al., 2010).
Exposure to sublethal concentration of ZEN significantly reduced T3, T4, and VTG content (Yang et al., 2021).
In a different fish species, male Rainbow Trout, zearalenone induced high sperm concentration and high plasma vitellogenin levels and induced early ovarian development in females (Woźny et al., 2020).
FUMONISINS
Almost no studies have been reported on other mycotoxins’ reproductive toxicity. However, Nile Tilapia fed a diet containing 2000 μg AFB1/kg diet for 24 weeks displayed an impaired reproductive performance characterized by a significant reduction in relative fecundity and gonadosomatic index (GSI) in females and significant differences in GSI, testosterone, milt count, and motility in males (Marijani et al., 2019).
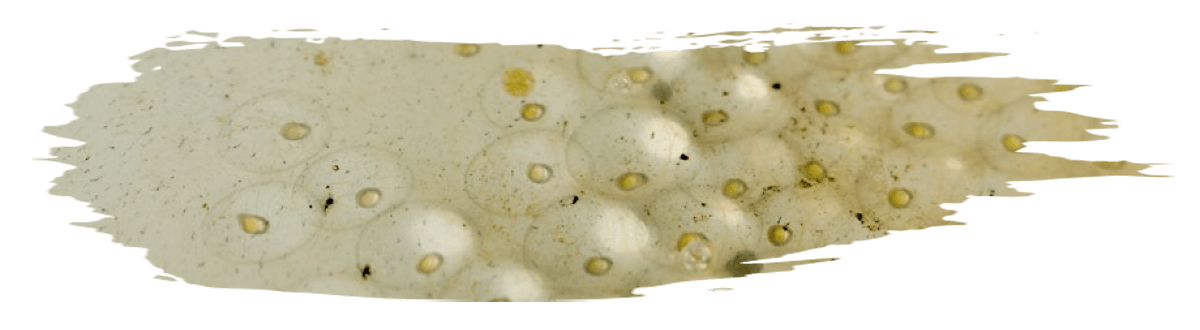
CONCLUSIONS
Mycotoxins are among the most potent cancer-causing agents. Ingestion through the diet can pose long-term health risks, including liver and kidney disease and immune system suppression.
Because the toxic effect of mycotoxins may be dependent on the type and quantity of mycotoxin content in the feed, but also on the duration of exposure, animal species, sex, and age, it is necessary to keep a standard to perform the tests on the duration of the experiments.
⇰ This review outlines an approach to the occurrence of mycotoxin-contaminated animal feed, as well as the toxic effects that mycotoxins may produce in fish and their residues in meat and organs.
The aflatoxins (B1, B2, G1, G2 and M1), ochratoxin A, and toxins produced by Fusarium molds (FB1, FB2, and FB3; trichothecenes like deoxynivalenol, T-2 and HT-2 toxin and ZEN) are the mycotoxins of most concern from a food safety perspective in animal products, but in studies with different species of fish, the AFBs are the mycotoxin most studied.
The consequences of mycotoxin contamination in fish feeding are similar to those found in other animal species intended for human consumption, and they are directly related to production losses, particularly reduced weight gain and feed conversion, but also immune impairment and organ lesions. Therefore, extreme caution is required in the production and storage of animal feed.
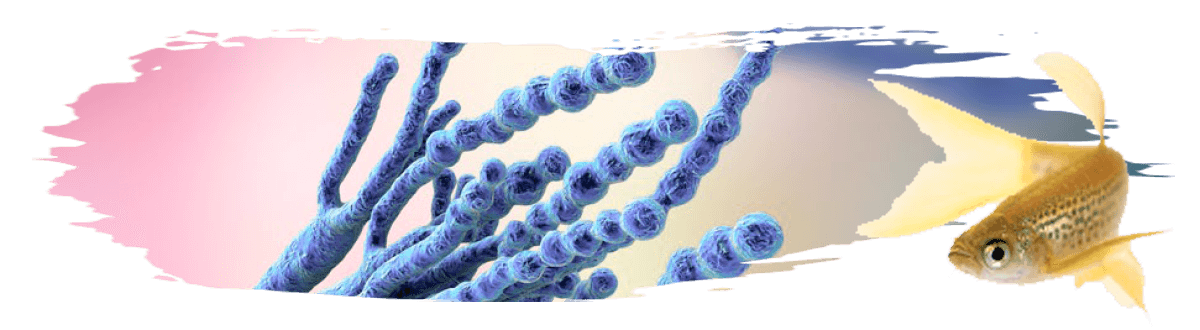
BIBLIOGRAPHY
Abdel-Tawwab, M., Khalifa, E., Diab, A.M., Khallaf, M.A., Abdel-Razek, N., Khalil, R.H., 2020. Dietary garlic and chitosan alleviated zearalenone toxic effects on performance, immunity, and challenge of European sea bass, Dicentrarchus labrax, to Vibrio alginolyticus infection. Aquaculture International 28, 493-510.
Abdel-Tawwab, M., Khalil, R.H., Diab, A.M., Khallaf, M.A., Abdel-Razek, N., Abdel-Latif, H.M., Khalifa, E., 2021. Dietary garlic and chitosan enhanced the antioxidant capacity, immunity, and modulated the transcription of HSP70 and Cytokine genes in Zearalenone-intoxicated European seabass. Fish & Shellfish Immunology 113, 35-41.
Abrar, M., Anjum, F.M., Butt, M.S., Pasha, I., Randhawa, M.A., Saeed, F., Waqas, K., 2013. Aflatoxins: biosynthesis, occurrence, toxicity, and remedies. Critical reviews in food science and nutrition 53, 862-874.
Adeyemo, B.T., Oloyede, T.L., Ogeh, A.V., Orkuma, C.J., 2016a. Growth performance and serum lipids profile of Clarias gariepinus catfish following experimental dietary exposure to fumonisin B1. Open Journal of Veterinary Medicine 6, 127-138.
Adeyemo, B.T., Tiamiyu, L.O., Ayuba, V.O., Cheikyula, J.O., 2016b. Effects of dietary fumonisin B1 on haematology and growth performance of the clariid fish Heterobranchus longifilis. J. Agric. Vet. Sci 9, 26-33.
Ahmad, A., Abdullah, S.R.S., Hasan, H.A., Othman, A.R., Ismail, N.I., 2021. Aquaculture industry: Supply and demand, best practices, effluent and its current issues and treatment technology. Journal of environmental management 287, 112271.
ALTUĞ, G., ÖZYURT, G., 2003. Level of aflatoxin in some fish feeds from fish farming processes, feed factories and imported feeds. Turkish Journal of Veterinary & Animal Sciences 27, 1247-1252.
Amuzie, C.J., Pestka, J.J., 2010. Suppression of insulin-like growth factor acid-labile subunit expression—A novel mechanism for deoxynivalenol- induced growth retardation. Toxicological Sciences 113, 412-421.
Arunachalam, C., Doohan, F.M., 2013. Trichothecene toxicity in eukaryotes: Cellular and molecular mechanisms in plants and animals. Toxicology letters 217, 149-158.
Asim, M., Sarma, M.P., Thayumanavan, L., Kar, P., 2011. Role of aflatoxin B1 as a risk for primary liver cancer in north Indian population. Clinical biochemistry 44, 1235-1240.
Ayala, A., Muñoz, M.F., Argüelles, S., 2014. Lipid peroxidation: production, metabolism, and signaling mechanisms of malondialdehyde and 4-hydroxy-2-nonenal. Oxidative medicine and cellular longevity 2014.
Ayyat, M.S., Ayyat, A.M.N., Al-Sagheer, A.A., El-Hais, A.E.-A.M., 2018. Effect of some safe feed additives on growth performance, blood biochemistry, and bioaccumulation of aflatoxin residues of Nile tilapia fed aflatoxin-B1 contaminated diet. Aquaculture 495, 27-34.
Bakos, K., Kovács, R., Staszny, Á., Sipos, D.K., Urbányi, B., Müller, F., Csenki, Z., Kovács, B., 2013. Developmental toxicity and estrogenic potency of zearalenone in zebrafish (Danio rerio). Aquatic Toxicology 136-137, 13-21.
Baldissera, M.D., Souza, C.F., da Silva, H.N.P., Henn, A.S., Duarte, F.A., da Costa, S.T., Da Silva, A.S., Baldisserotto, B., 2020a. Diphenyl diselenide modulates splenic purinergic signaling in silver catfish fed diets contaminated with fumonisin B1: An attempt to improve immune and hemostatic responses. Comparative Biochemistry and Physiology Part C: Toxicology & Pharmacology 227, 108624.
Baldissera, M.D., Souza, C.F., da Silva, H.N.P., Zeppenfeld, C.C., Dornelles, J.L., Henn, A.S., Duarte, F.A., da Costa, S.T., Da Silva, A.S., Cunha, M.A., 2020b. Diphenyl diselenide dietary supplementation protects against fumonisin B1-induced oxidative stress in brains of the silver catfish Rhamdia quelen. Comparative Biochemistry and Physiology Part C: Toxicology & Pharmacology 231, 108738.
Baldissera, M.D., Souza, C.F., da Silva, J.A., Barroso, D.C., Glória, E.M., Mesadri, J., Wagner, R., Baldisserotto, B., Val, A.L., 2020c. Dietary ochratoxin A (OTA) decreases growth performance and impairs muscle antioxidant system and meat fatty acid profiles in juvenile tambaqui (Colossoma macropomum). Comparative Biochemistry and Physiology Part C: Toxicology & Pharmacology 236, 108803.
Bautista, M.N., Lavilla‐Pitogo, C.R., Subosa, P.F., Begino, E.T., 1994. Aflatoxin B1 contamination of shrimp feeds and its effect on growth and hepatopancreas of pre‐adult Penaeus monodon. Journal of the Science of Food and Agriculture 65, 5-11. Benkerroum, N., 2020. Chronic and acute toxicities of aflatoxins: Mechanisms of action. International journal of environmental research and public health 17, 423.
Bernhoft, A., Høgåsen, H.R., Rosenlund, G., Moldal, T., Grove, S., Berntssen, M.H., Thoresen, S.I., Alexander, J., 2018. Effects of dietary deoxynivalenol or ochratoxin A on performance and selected health indices in Atlantic salmon (Salmo salar). Food and Chemical Toxicology 121, 374-386.
Bintvihok, A., Ponpornpisit, A., Tangtrongpiros, J., Panichkriangkrai, W., Rattanapanee, R., Doi, K., Kumagai, S., 2003. Aflatoxin contamination in shrimp feed and effects of aflatoxin addition to feed on shrimp production. Journal of food protection 66, 882-885.
Chen, C., Wu, F., 2017. The need to revisit ochratoxin A risk in light of diabetes, obesity, and chronic kidney disease prevalence. Food and Chemical Toxicology 103, 79-85.
Chen, J., Wei, Z., Wang, Y., Long, M., Wu, W., Kuca, K., 2021. Fumonisin B1: Mechanisms of toxicity and biological detoxification progress in animals. Food and chemical toxicology 149, 111977.
Creppy, E., Størmer, F., Kern, D., Röschenthaler, R., Dirheimer, G., 1983. Effects of ochratoxin A metabolites on yeast phenylalanyl-tRNA synthetase and on the growth and in vivo protein synthesis of hepatoma cells. Chemico-biological interactions 47, 239-247.
da Rocha, M.E.B., Freire, F.d.C.O., Maia, F.E.F., Guedes, M.I.F., Rondina, D., 2014. Mycotoxins and their effects on human and animal health. Food Control 36, 159-165.
da Silva, S.C.C., Lala, B., de Oliveira Carniatto, C.H., Schamber, C.R., Nascimento, C.S., Braccini, G.L., Porto, C., Roldi, G., Tanamati, F., Gasparino, E., 2019. Fumonisin affects performance and modulates the gene expression of IGF-1 and GHR in Nile tilapia fingerlings and juveniles. Aquaculture 507, 233-237.
De Boevre, M., Di Mavungu, J.D., Landschoot, S., Audenaert, K., Eeckhout, M., Maene, P., Haesaert, G., De Saeger, S., 2012. Natural occurrence of mycotoxins and their masked forms in food and feed products. World Mycotoxin Journal 5, 207-219.
Deng, Y., Wang, Y., Deng, Q., Sun, L., Wang, R., Ye, L., Tao, S., Liao, J., Gooneratne, R., 2021. Fungal diversity and mycotoxin contamination in dried fish products in Zhanjiang market, China. Food control 121, 107614.
Dhanasekaran, D., Shanmugapriya, S., Thajuddin, N., Panneerselvam, A., 2011. Aflatoxins and aflatoxicosis in human and animals. Aflatoxins- Biochemistry and Molecular Biology 10, 221-254.
Duarte, S.C., Lino, C.M., Pena, A., 2012. Food safety implications of ochratoxin A in animal-derived food products. The Veterinary Journal 192, 286-292.
Edite Bezerra da Rocha, M., Freire, F.d.C.O., Erlan Feitosa Maia, F., Izabel Florindo Guedes, M., Rondina, D., 2014. Mycotoxins and their effects on human and animal health. Food Control 36, 159-165.
Fegan, D., Spring, P. 2007. Recognizing the reality of the aquaculture mycotoxin problem: searching for a common and effective solution. In: Nutritional biotechnology in the feed and food industries: Proceedings of Alltech’s 23rd Annual Symposium. The new energy crisis: food, feed or fuel?, 343-354.
Gan, F., Hou, L., Xu, H., Liu, Y., Chen, X., Huang, K., 2022. PCV2 infection aggravates OTA-induced immunotoxicity in vivo and in vitro. Ecotoxicology and Environmental Safety 235, 113447.
Ghafarifarsani, H., Kachuei, R., Imani, A., 2021. Dietary supplementation of garden thyme essential oil ameliorated the deteriorative effects of aflatoxin B1 on growth performance and intestinal inflammatory status of rainbow trout (Oncorhynchus mykiss). Aquaculture 531, 735928.
Gonçalves, R.A., Naehrer, K., Santos, G.A., 2018. Occurrence of mycotoxins in commercial aquafeeds in Asia and Europe: a real risk to aquaculture? Reviews in Aquaculture 10, 263-280.
Greco, M., Pardo, A., Pose, G., 2015. Mycotoxigenic fungi and natural co-occurrence of mycotoxins in rainbow trout (Oncorhynchus mykiss) feeds. Toxins 7, 4595-4609.
HASSANPOUR, S., Sarvi Moghanlou, K., Razi, M., Imani, A., 2021. Alterations in growth indices and body composition of goldfish (Carassius auratus) fed on diets contaminated with different levels of Aflatoxin B1 and Zearalenone toxins. Journal of Fisheries 74, 153-163.
Hassen, W., Abid, S., Achour, A., Creppy, E., Bacha, H., 2004. Ochratoxin A and β2-microglobulinuria in healthy individuals and in chronic interstitial nephropathy patients in the centre of Tunisia: a hot spot of Ochratoxin A exposure. Toxicology 199, 185-193.
Hooft, J.M., Encarnação, P., Bureau, D.P., 2011. Rainbow trout (Oncorhynchus mykiss) is extremely sensitive to the feed-borne Fusarium mycotoxin deoxynivalenol (DON). Aquaculture 311, 224-232.
Hooft, J.M., Ferreira, C., Lumsden, J.S., Sulyok, M., Krska, R., Bureau, D.P., 2019. The effects of naturally occurring or purified deoxynivalenol (DON) on growth performance, nutrient utilization and histopathology of rainbow trout (Oncorhynchus mykiss). Aquaculture 505, 319-332.
Hope, J.H., Hope, B.E., 2012. A review of the diagnosis and treatment of Ochratoxin A inhalational exposure associated with human illness and kidney disease including focal segmental glomerulosclerosis. Journal of Environmental and Public Health 2012.
Jackson, A., 2012. Fishmeal and fish oil and its role in sustainable aquaculture. International aquafeed 15, 18-21. Jørgensen, K., Petersen, A., 2002. Content of ochratoxin A in paired kidney and meat samples from healthy Danish slaughter pigs. Food Additives & Contaminants 19, 562-567.
Juraschek, L.M., Kappenberg, A., Amelung, W., 2022. Mycotoxins in soil and environment. The Science of the total environment 814, 152425. Kar, S., Sangem, P., Anusha, N., Senthilkumaran, B., 2021. Endocrine disruptors in teleosts: Evaluating environmental risks and biomarkers. Aquaculture and Fisheries 6, 1-26.
Khalafalla, M.M., Zayed, N.F., Amer, A.A., Soliman, A.A., Zaineldin, A.I., Gewaily, M.S., Hassan, A.M., Van Doan, H., Tapingkae, W., Dawood, M.A., 2022. Dietary Lactobacillus acidophilus ATCC 4356 Relieves the Impacts of Aflatoxin B1 Toxicity on the Growth Performance, Hepatorenal Functions, and Antioxidative Capacity of Thinlip Grey Mullet (Liza ramada)(Risso 1826). Probiotics and Antimicrobial Proteins 14, 189-203.
Koletsi, P., Schrama, J.W., Graat, E.A., Wiegertjes, G.F., Lyons, P., Pietsch, C., 2021. The Occurrence of Mycotoxins in Raw Materials and Fish Feeds in Europe and the Potential Effects of Deoxynivalenol (DON) on the Health and Growth of Farmed Fish Species—A Review. Toxins 13, 403.
Lala, B., Santos, C., Roldi, G., Roça, R., Sampaio, G., Garcia, A., Garrido, B., Ricci, G., Refundini, G., Braccini, G., 2021. Fumonisins alter redox balance in Nile tilapia fingerlings. Aquaculture 530, 735735.
Li, J., Wang, Y., Deng, Y., Wang, X., Wu, W., Nepovimova, E., Wu, Q., Kuca, K., 2022. Toxic mechanisms of the trichothecenes T-2 toxin and deoxynivalenol on protein synthesis. Food and Chemical Toxicology, 113044.
Limonciel, A., Jennings, P., 2014. A review of the evidence that ochratoxin A is an Nrf2 inhibitor: implications for nephrotoxicity and renal carcinogenicity. Toxins 6, 371-379. Liu, X., Wu, P., Jiang, W.-D., Liu, Y., Jiang, J., Kuang, S.-Y., Tang, L., Zhou, X.-Q., Feng, L., 2020. Effects of dietary ochratoxin a on growth performance and intestinal apical junctional complex of juvenile grass carp (Ctenopharyngodon idella). Toxins 13, 11.
Longobardi, C., Damiano, S., Andretta, E., Prisco, F., Russo, V., Pagnini, F., Florio, S., Ciarcia, R., 2021. Curcumin Modulates Nitrosative Stress, Inflammation and DNA Damage and Protects against Ochratoxin A-Induced Hepatotoxicity and Nephrotoxicity in Rats. Antioxidants 10, 1239.
Magouz, F., Abu-Ghanima, H., Zaineldin, A.I., Gewaily, M.S., Soliman, A., Amer, A.A., Moustafa, E.M., Younis, E.M., Abdel-Warith, A.-W.A., Davies, S.J., 2022. Dietary Bacillus subtilis relieved the growth retardation, hepatic failure, and antioxidative depression induced by ochratoxin A in Thinlip Mullet (Liza ramada). Aquaculture Reports 22, 100984.
Mahfouz, M., 2015. Ameliorative effect of curcumin on aflatoxin B1-induced changes in liver gene expression of Oreochromis niloticus. Molecular Biology 49, 275-286.
Mansour, A., Soliman, M., Srour, T., Nour, A., 2016. EVALUATE THE ABILITY OF DIETARY ACTIVE YEAST TO ALLEVIATE NEGATIVE EFFECT OF OCHRATOXIN A (OTA) ON NILE TILAPIA (OREOCHROMIS NILOTICUS) FINGERLINGS. Egyptian Journal of Nutrition and Feeds 19, 147-159.
Mansour, T., Omar, A., Soliman, K., Srour, T., Nour, A., 2015. The antagonistic effect of whey on ochratoxin a toxicity on the growth performance, feed utilization, liver and kidney functions of Nile tilapia (Oreochromis niloticus). Middle East. J. Appl. Sci 5, 176-183.
Marijani, E., Charo-Karisa, H., Gnonlonfin, G.J.B., Kigadye, E., Okoth, S., 2019. Effects of aflatoxin B1 on reproductive performance of farmed Nile tilapia. International Journal of Veterinary Science and Medicine 7, 35-42.
Marijani, E., Wainaina, J.M., Charo-Karisa, H., Nzayisenga, L., Munguti, J., Gnonlonfin, G.J.B., Kigadye, E., Okoth, S., 2017. Mycoflora and mycotoxins in finished fish feed and feed ingredients from smallholder farms in East Africa. The Egyptian Journal of Aquatic Research 43, 169-176.
Marroquín-Cardona, A., Johnson, N., Phillips, T., Hayes, A., 2014. Mycotoxins in a changing global environment–a review. Food and Chemical Toxicology 69, 220-230.
Matejova, I., Faldyna, M., Modra, H., Blahova, J., Palikova, M., Markova, Z., Franc, A., Vicenova, M., Vojtek, L., Bartonkova, J., 2017a. Effect of T-2 toxin-contaminated diet on common carp (Cyprinus carpio L.). Fish & shellfish immunology 60, 458-465.
Matejova, I., Svobodova, Z., Vakula, J., Mares, J., Modra, H., 2017b. Impact of mycotoxins on aquaculture fish species: a review. Journal of the world aquaculture society 48, 186-200.
Modra, H., Palikova, M., Hyrsl, P., Bartonkova, J., Papezikova, I., Svobodova, Z., Blahova, J., Mares, J., 2020. Effects of trichothecene mycotoxin T-2 toxin on haematological and immunological parameters of rainbow trout (Oncorhynchus mykiss). Mycotoxin research 36, 319-326.
Modra, H., Sisperova, E., Blahova, J., Enevova, V., Fictum, P., Franc, A., Mares, J., Svobodova, Z., 2018. Elevated concentrations of T‐2 toxin cause oxidative stress in the rainbow trout (Oncorhynchus mykiss). Aquaculture Nutrition 24, 842-849.
Muthulakshmi, S., Hamideh, P.F., Habibi, H.R., Maharajan, K., Kadirvelu, K., Mudili, V., 2018a. Mycotoxin zearalenone induced gonadal impairment and altered gene expression in the hypothalamic–pituitary–gonadal axis of adult female zebrafish (Danio rerio). Journal of Applied Toxicology 38, 1388-1397.
Muthulakshmi, S., Maharajan, K., Habibi, H.R., Kadirvelu, K., Venkataramana, M., 2018b. Zearalenone induced embryo and neurotoxicity in zebrafish model (Danio rerio): Role of oxidative stress revealed by a multi biomarker study. Chemosphere 198, 111-121.
Mwihia, E.W., Lyche, J.L., Mbuthia, P.G., Ivanova, L., Uhlig, S., Gathumbi, J.K., Maina, J.G., Eshitera, E.E., Eriksen, G.S., 2020. Co-Occurrence and levels of mycotoxins in fish feeds in Kenya. Toxins 12, 627.
Nácher-Mestre, J., Serrano, R., Beltrán, E., Pérez-Sánchez, J., Silva, J., Karalazos, V., Hernandez, F., Berntssen, M.H., 2015. Occurrence and potential transfer of mycotoxins in gilthead sea bream and Atlantic salmon by use of novel alternative feed ingredients. Chemosphere 128, 314-320.
Naiel, M.A., Ismael, N.E., Shehata, S.A., 2019. Ameliorative effect of diets supplemented with rosemary (Rosmarinus officinalis) on aflatoxin B1 toxicity in terms of the performance, liver histopathology, immunity and antioxidant activity of Nile Tilapia (Oreochromis niloticus). Aquaculture 511, 734264.
Oliveira, M., Vasconcelos, V., 2020. Occurrence of mycotoxins in fish feed and its effects: A review. Toxins 12, 160. Omotayo, O.P., Omotayo, A.O., Mwanza, M., Babalola, O.O., 2019. Prevalence of mycotoxins and their consequences on human health. Toxicological research 35, 1-7.
Patriarca, A., Pinto, V.F., 2017. Prevalence of mycotoxins in foods and decontamination. Current Opinion in Food Science 14, 50-60.
Peng, K., Chen, B., Zhao, H., Huang, W., 2021. Toxic Effects of Aflatoxin B1 in Chinese Sea Bass (Lateolabrax maculatus). Toxins 13, 844.
Pietsch, C., 2020. Risk assessment for mycotoxin contamination in fish feeds in Europe. Mycotoxin research 36, 41-62.
Pietsch, C., Burkhardt-Holm, P., 2015. Feed-borne exposure to deoxynivalenol leads to acute and chronic effects on liver enzymes and histology in carp. World Mycotoxin Journal 8, 619-627.
Pietsch, C., Katzenback, B.A., Garcia-Garcia, E., Schulz, C., Belosevic, M., Burkhardt-Holm, P., 2015a. Acute and subchronic effects on immune responses of carp (Cyprinus carpio L.) after exposure to deoxynivalenol (DON) in feed. Mycotoxin research 31, 151-164.
Pietsch, C., Kersten, S., Burkhardt-Holm, P., Valenta, H., Dänicke, S., 2013. Occurrence of deoxynivalenol and zearalenone in commercial fish feed: an initial study. Toxins (Basel) 5, 184-192.
Pietsch, C., Kersten, S., Valenta, H., Dänicke, S., Schulz, C., Burkhardt-Holm, P., Junge, R., 2015b. Effects of dietary exposure to zearalenone (ZEN) on carp (Cyprinus carpio L.). Toxins 7, 3465 3480.
Proctor, R.H., McCormick, S.P., Kim, H.-S., Cardoza, R.E., Stanley, A.M., Lindo, L., Kelly, A., Brown, D.W., Lee, T., Vaughan, M.M., 2018. Evolution of structural diversity of trichothecenes, a family of toxins produced by plant pathogenic and entomopathogenic fungi. PLoS pathogens 14, e1006946.
Pumipuntu, N., Tengjaroenkul, B., Pimpukdee, K., 2013. Effects of diet containing aflatoxin B1 and fumonisin B1 on hematology, immune response and histopathology in nile tilapia. KKU Veterinary Journal (Thailand).
Rahimtula, A., Chong, X., 1991. Alterations in calcium homeostasis as a possible cause of ochratoxin A nephrotoxicity. IARC Scientific Publications, 207-214.
Rogowska, A., Pomastowski, P., Sagandykova, G., Buszewski, B., 2019. Zearalenone and its metabolites: Effect on human health, metabolism and neutralisation methods. Toxicon 162, 46-56.
Rushing, B.R., Selim, M.I., 2017. Structure and oxidation of pyrrole adducts formed between aflatoxin B2a and biological amines. Chemical research in toxicology 30, 1275-1285.
Schwartz, P., Thorpe, K.L., Bucheli, T.D., Wettstein, F.E., Burkhardt-Holm, P., 2010. Short-term exposure to the environmentally relevant estrogenic mycotoxin zearalenone impairs reproduction in fish. Science of The Total Environment 409, 326-333.
Scott, P., 2012. Recent research on fumonisins: a review. Food additives & contaminants: part A 29, 242-248.
Shalaby, A.M., Abbassa, A.H., 2009. The opposing effect of ascorbic acid (vitamin C) on ochratoxin toxicity in Nile tilapia (Oreochromis niloticus). Acta Polenica 2, 18-22.
Shin, H.S., Lee, H.J., Pyo, M.C., Ryu, D., Lee, K.-W., 2019. Ochratoxin A-induced hepatotoxicity through phase I and phase II reactions regulated by AhR in liver cells. Toxins 11, 377.
Stiborová, M., Arlt, V.M., Schmeiser, H.H., 2016. Balkan endemic nephropathy: an update on its aetiology. Archives of toxicology 90, 2595-2615.
Stoev, S.D., 2022. Studies on teratogenic effect of ochratoxin A given via mouldy diet in mice in various sensitive periods of the pregnancy and the putative protection of phenylalanine. Toxicon 210, 32-38.
Tacon, A.G., Hasan, M.R., Metian, M., 2011. Demand and supply of feed ingredients for farmed fish and crustaceans: trends and prospects. FAO Fisheries and Aquaculture technical paper, I.
Tacon, A.G., Metian, M., 2008. Aquaculture feed and food safety: the role of the food and agriculture organization and the Codex Alimentarius. Annals of the New York Academy of Sciences 1140, 50-59.
Taheur, F.B., Fedhila, K., Chaieb, K., Kouidhi, B., Bakhrouf, A., Abrunhosa, L., 2017. Adsorption of aflatoxin B1, zearalenone and ochratoxin A by microorganisms isolated from Kefir grains. International journal of food microbiology 251, 1-7.
Tola, S., Bureau, D.P., Hooft, J.M., Beamish, F.W., Sulyok, M., Krska, R., Encarnação, P., Petkam, R., 2015. Effects of wheat naturally contaminated with Fusarium mycotoxins on growth performance and selected health indices of red tilapia (Oreochromis niloticus× O. mossambicus). Toxins 7, 1929-1944.
Varga, J., Frisvad, J.C., Samson, R., 2011. Two new aflatoxin producing species, and an overview of Aspergillus section Flavi. Studies in mycology 69, 57-80.
Vila-Donat, P., Marín, S., Sanchis, V., Ramos, A., 2018. A review of the mycotoxin adsorbing agents, with an emphasis on their multi-binding capacity, for animal feed decontamination. Food and chemical toxicology 114, 246-259.
Voss, K.A., Riley, R.T., 2013. Fumonisin toxicity and mechanism of action: Overview and current perspectives. Food Safety 1, 2013006-2013006.
Wang, X., Liu, Q., Ihsan, A., Huang, L., Dai, M., Hao, H., Cheng, G., Liu, Z., Wang, Y., Yuan, Z., 2012. JAK/STAT pathway plays a critical role in the proinflammatory gene expression and apoptosis of RAW264. 7 cells induced by trichothecenes as DON and T-2 toxin. Toxicological Sciences 127, 412-424.
Wang, X., Wu, Q., Wan, D., Liu, Q., Chen, D., Liu, Z., Martínez-Larrañaga, M.R., Martínez, M.A., Anadón, A., Yuan, Z., 2016. Fumonisins: Oxidative stress-mediated toxicity and metabolism in vivo and in vitro. Archives of toxicology 90, 81-101.
Woźny, M., Dobosz, S., Hliwa, P., Gomułka, P., Król, J., Obremski, K., Blahova, J., Svobodova, Z., Michalik, O., Ocalewicz, K., 2020. Feed-borne exposure to zearalenone impairs reproduction of rainbow trout. Aquaculture 528, 735522.
Woźny, M., Obremski, K., Hliwa, P., Gomułka, P., Różyński, R., Wojtacha, P., Florczyk, M., Segner, H., Brzuzan, P., 2019. Feed contamination with zearalenone promotes growth but affects the immune system of rainbow trout. Fish & shellfish immunology 84, 680-694.
Woźny, M., Obremski, K., Jakimiuk, E., Gusiatin, M., Brzuzan, P., 2013. Zearalenone contamination in rainbow trout farms in north-eastern Poland. Aquaculture 416, 209-211.
Wu, J., Tu, D., Yuan, L.-Y., Yi, J.-e., Tian, Y., 2015. T-2 toxin regulates steroid hormone secretion of rat ovarian granulosa cells through cAMP-PKA pathway. Toxicology letters 232, 573-579.
Yang, G., Wang, Y., Wang, T., Wang, D., Weng, H., Wang, Q., Chen, C., 2021. Variations of enzymatic activity and gene expression in zebrafish (Danio rerio) embryos co-exposed to zearalenone and fumonisin B1. Ecotoxicology and Environmental Safety 222, 112533.
Yang, S., Wang, Y., Beier, R.C., Zhang, H., Ruyck, K.D., Sun, F., Cao, X., Shen, J., Zhang, S., Wang, Z., 2015. Simultaneous determination of type A and B trichothecenes and their main metabolites in food animal tissues by ultraperformance liquid chromatography coupled with triple-quadrupole mass spectrometry. Journal of agricultural and food chemistry 63, 8592-8600.
Zahran, E., Elbahnaswy, S., Mamdouh, A.Z., El‐Matbouli, M., 2021. Xenosteroids in aquaculture with special consideration to Lake Manzala (Northern delta lake, Egypt): Types, sources and mechanism of action. Aquaculture Research 52, 5962-5977.
Zahran, E., Manning, B., Seo, J.-K., Noga, E.J., 2016. The effect of Ochratoxin A on antimicrobial polypeptide expression and resistance to water mold infection in channel catfish (Ictalurus punctatus). Fish & Shellfish Immunology 57, 60-67.
Zahran, E., Risha, E., Hamed, M., Ibrahim, T., Palić, D., 2020. Dietary mycotoxicosis prevention with modified zeolite (Clinoptilolite) feed additive in Nile tilapia (Oreochromis niloticus). Aquaculture 515, 734562.
Zhang, H.-Y., Wang, Y.-L., Zhou, X.-Q., Jiang, W.-D., Wu, P., Liu, Y., Zhang, L., Mi, H.-F., Jiang, J., Kuang, S.-Y., 2021. Zearalenone induces immuno- compromised status via TOR/NF/κB pathway and aggravates the spread of Aeromonas hydrophila to grass carp gut (Ctenopharyngodon idella). Ecotoxicology and Environmental Safety 225, 112786.